Key takeaways
~ Glutamate is the most abundant neurotransmitter in the brain and periphery.
~ Cells can make glutamate from glutamine or alpha-ketoglutarate.
~ There are genetic variants that impact glutamate levels a bit, but overall, glutamate levels are tightly controlled by multiple pathways.
~ Altered glutamate signaling is implicated in schizophrenia, OCD, and migraines.
Members will see their genotype report below, plus additional solutions in the Lifehacks section. Consider joining today.
What is glutamate?
Glutamate is the major excitatory neurotransmitter in the central nervous system (CNS). It’s important for learning, memory, and mood, but it’s not as well known as other neurotransmitters like dopamine or serotonin.
As an excitatory neurotransmitter, glutamate is essential for learning, attention, and focus – but too much glutamate causes too much stimulation in the brain. A balance between stimulation and inhibition is needed.
Let’s look at the research on how glutamate is synthesized, what receptors it binds to, and why it is so important for cognitive function.
How glutamate is synthesized:
Glutamate is the most abundant free amino acid in the brain.[ref] As an excitatory amino acid, glutamate levels are strictly controlled by several mechanisms. Glutamate can be synthesized from multiple sources and can also be converted into other neurotransmitters or amino acids. These pathways interact to keep glutamate levels at the right balance.
Here’s a graphical overview of the synthesis pathways:
Glutamate from glutamine:
Glutamate can be synthesized from the amino acid glutamine with the help of the enzyme glutaminase (GLS and GLS2 genes). This conversion releases a molecule of ammonia (NH3). In neurons, glutamate is then packaged into synaptic vesicles by vesicular glutamate transporters (VGLUTs) and stored in the presynaptic terminal before release.
The conversion of glutamine to glutamate is a two-way street. Glutamate can also be converted to glutamine with the addition of a molecule of ammonia (NH3).
Glutamate + ATP + NH3 → Glutamine + ADP + phosphate
Glutamine is the most abundant free amino acid in the body. In addition to being used to synthesize glutamate, glutamine is incorporated into many proteins and can be used for nucleotide synthesis. It is considered “conditionally essential,” meaning that most of the time the body can make enough glutamine, but during times of stress (illness, etc.) the demand for glutamine may be such that it is needed from food or supplements.[ref]
Glutamate from alpha-ketoglutarate:
Glutamate can also be converted to α-ketoglutarate using the glutamate dehydrogenase genes (GLUD1, GLUD2) and releasing ammonia in the process. This synthesis of alpha-ketoglutarate feeds into the Krebs (citric acid, TCA) cycle for the production of ATP.
In the liver, this process is also a two-way street and alpha-ketoglutarate can be converted to glutamate.[ref]
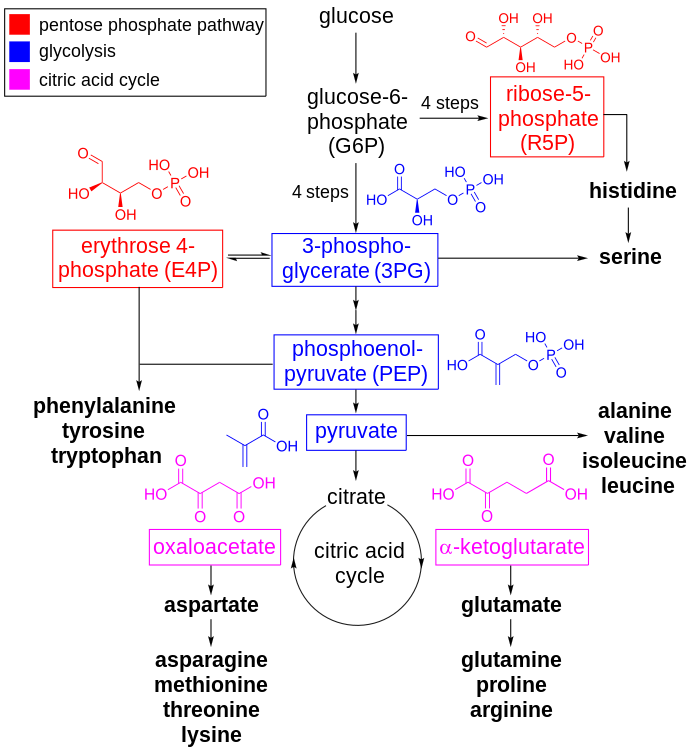
Glutamate is also the precursor for GABA, the main inhibitory neurotransmitter. This balance between glutamate and GABA is key to how the brain works, balancing excitation with inhibition. An imbalance between glutamate and GABA can lead to neuropathological disorders. Glutamate is converted to GABA by the enzymes GAD1 and GAD2.
What does glutamate do in the brain?
Glutamate is an excitatory neurotransmitter, which means that it causes neurons to fire and pass a message along to the next cell.
Glutamate is released into the synaptic cleft and taken up by glutamate receptors. Transporters tightly regulate the amount of glutamate that is released by neurons, astrocytes, and other cells in the brain. These transporters are called excitatory amino acid transporters (EAATs).
In addition to being synthesized and released by neurons, glutamate is also taken up by astrocytes. Astrocytes help regulate the amount of glutamate in the synaptic cleft, the space between one neuron and the next.
Glutamate causes action by binding to and activating receptors. Most cells in the brain and CNS have a glutamate receptor of one type or another. Glutamatergic neurons are neurons that make and release glutamate, but other types of neurons, such as dopaminergic and cholinergic neurons, can also have glutamate receptors.[ref]
There are two main types of glutamate receptors:[ref]
- Ionotropic receptors: AMPA, NMDA, and kainite receptors which are fast, excitatory receptors
- Metabotropic receptors: G-protein coupled receptors that modulate the release of glutamate and other neurotransmitters as well as being involved in synaptic plasticity
Binding to the various glutamate receptors then causes a variety of actions in the neuron.
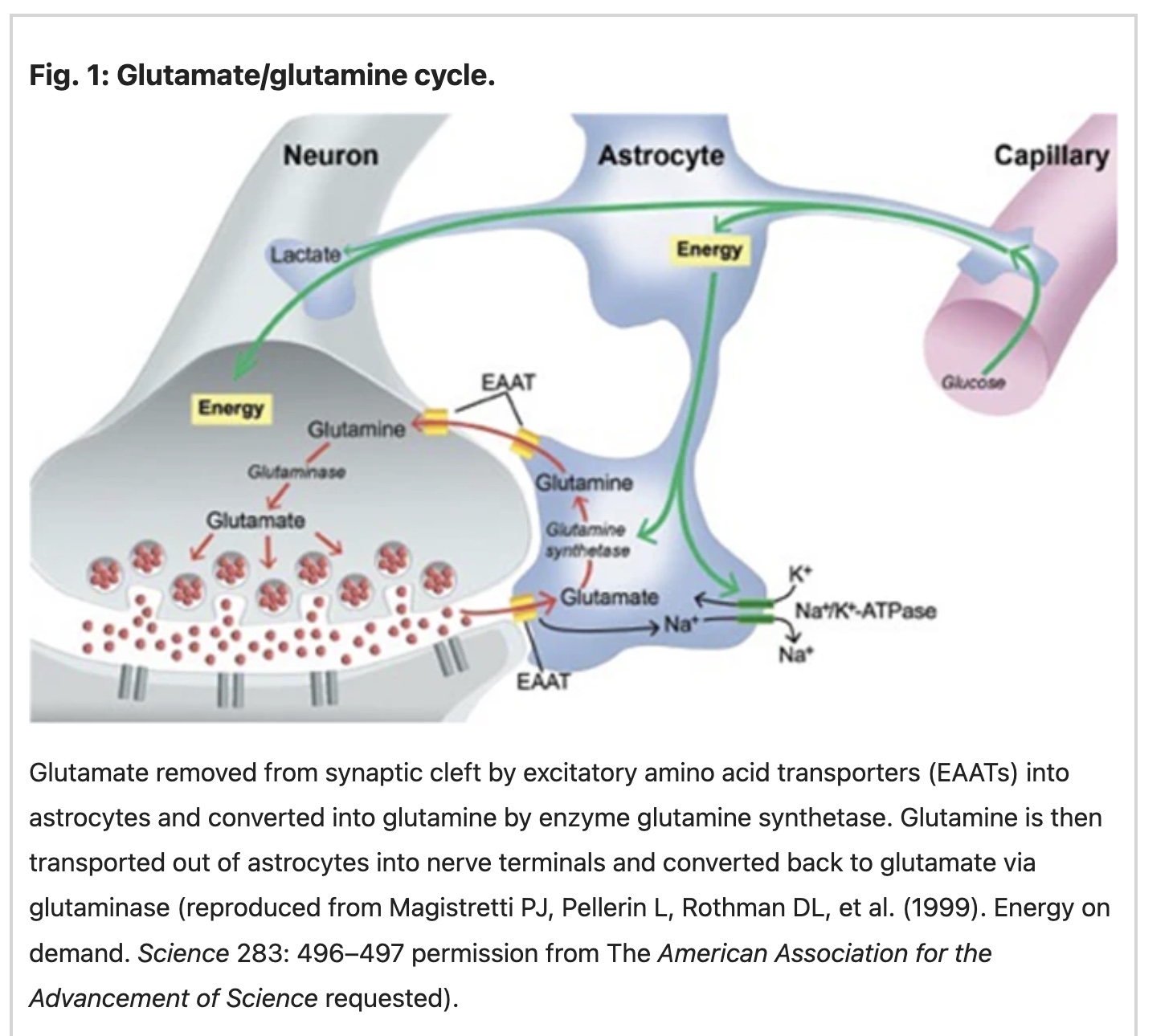
Glutamate levels in the brain are tightly controlled. After glutamate activates its receptors, it is rapidly removed from the synaptic cleft between neurons by glutamate transporters (EAATs) located on neurons and astrocytes. This regulates the amount of glutamate and prevents excitotoxicity. In astrocytes, glutamate is converted to glutamine by glutamine synthase. It is then transported back to the neurons for recycling into glutamate.[ref]
What happens when there is too much or too little glutamate in the brain?
Glutamate is essential in the brain, and different regions of the brain need different amounts. The receptors, feedback loops, astrocytes, and inflammation all play a role in glutamate levels.
Too much glutamate:
Excess glutamate from microglial cells is thought to cause neuroinflammation and depression. Microglia are like immune system cells in the brain that can respond to pathogens or damaged cells. They can engulf pathogens or damaged cells, or they can increase the expression of inflammatory cytokines. Microglia can synthesize glutamate from glutamine, thereby increasing glutamate levels in the brain. Microglia use the enzyme GLS1 (glutaminase 1) to convert glutamine to glutamate. Blocking GLS1 improves neuroinflammation (multiple sclerosis, HIV, brain infections) in animal studies. [ref]
It’s not as simple, though, as glutamate = bad.
Glutamate imbalance in autism:
In autism spectrum disorder, reduced glutamate levels are found in the striatum. Animal research shows that decreasing GLS1 (glutaminase enzyme) causes low glutamate in the forebrain and causes autism-like behavior (in mice).[ref] It’s not as simple as low glutamate causing autism. An imbalance between excitatory and inhibitory neurotransmission in different regions of the brain may occur in autism. Imaging studies show that glutamate/glutamine is higher in the primary sensorimotor cortex in autism spectrum disorder. Researchers concluded that this is associated with higher sensory responses, such as to sounds or tactile stimuli, in people with ASD..[ref]
OCD (obsessive-compulsive disorder) and glutamate balance:
Glutamate levels, or more specifically the balance of glutamate in certain regions of the brain, are also thought to play a role in OCD. Studies also show that people with OCD are likely to have higher levels of glutamate in their cerebrospinal fluid (CSF).[ref] Genetics comes into play with OCD. You’ll see in the genotype report section that genetic variants in the excitatory amino acid transporters are linked to susceptibility to OCD.
GABA levels:
Glutamate is the precursor for GABA, the main inhibitory neurotransmitter. GABA is like the “stop” while glutamate is the “go”. Too little conversion to GABA could mean too much glutamate. Does this play a role in depression? A new study shows that the ratio of GABA to glutamate does not play a role in depression. The study looked at patients with major depressive disorder before and after taking an antidepressant (or placebo). The results showed no relationship between changes in GABA and glutamate from the antidepressant related to changes in depression.[ref]
Seizures and excess glutamate:
Glutamate excitotoxicity is key to epileptic seizures. Studies show that glutamate levels are higher in certain brain regions just before or during seizures. Chronic seizures alter glutamate receptors and transporters, adding to the brain changes in epilepsy.[ref]
Addiction:
In addition to dopamine and other neurotransmitters, glutamate levels and glutamate receptors play a role in addiction. In chronic alcohol use, glutamate levels are elevated in the brain.[ref] One hypothesis of addiction involves an imbalance of glutamate and subsequent changes in neuroplasticity.[ref] In nicotine addiction, there is an increase in glutamate receptors (NMDA and AMPA) and more glutamate available at the synapse.[ref]
Essential tremor:
People with essential tremors have higher glutamate levels and lower levels of glutamine and several other amino acids. The imbalance of glutamate to GABA is thought to play a key role in the neurochemical oscillations in essential tremor.[ref]
Role of glutamate in migraines:
People with migraines generally have higher glutamate levels in their plasma and cerebrospinal fluid. Migraines activate the trigeminal nerve. Glutaminergic receptors on the trigeminal nerve are thought to play a role in migraine pain and in the spread of cortical depression. Too much glutamate is excitotoxic and can cause neuroinflammation and oxidative stress. This may be how glutamate plays a role in migraine (and why MSG is a migraine trigger for some people).[ref]
Role of glutamate in schizophrenia:
Researchers have known for some time that glutamate plays a significant role in schizophrenia, but it’s not clear whether it is due to excess glutamate, dysfunction of the NMDA receptor, or dysregulation in specific brain regions. Medications for schizophrenia generally either boost NMDA receptor function or block the dopamine receptor.[ref]
Glutamate interacts with the immune system:
Glial cells in the brain are part of the support network for neurons, and certain types of glial cells, including astrocytes and microglia, are responsible for immune responses in the brain.
Astrocytes can take up and release glutamate. As part of the brain’s immune response, astrocytes can release inflammatory cytokines in response to pathogens, stroke, or other injuries that cause neuroinflammation. When astrocytes are activated to be pro-inflammatory, they don’t take up as much glutamate from the synapse between neurons. This results in increased glutamate in the synaptic cleft during times of neuroinflammation.[ref]
One theory of researchers is that neuroinflammation has a role in addiction. For example, meth causes an inflammasome activation. The inflammatory activation then increases glutamate by altering the way astrocytes regulate glutamate uptake and glutamine release. The increased glutamate then upregulates the glutamate receptors and rewires the brain.[ref]
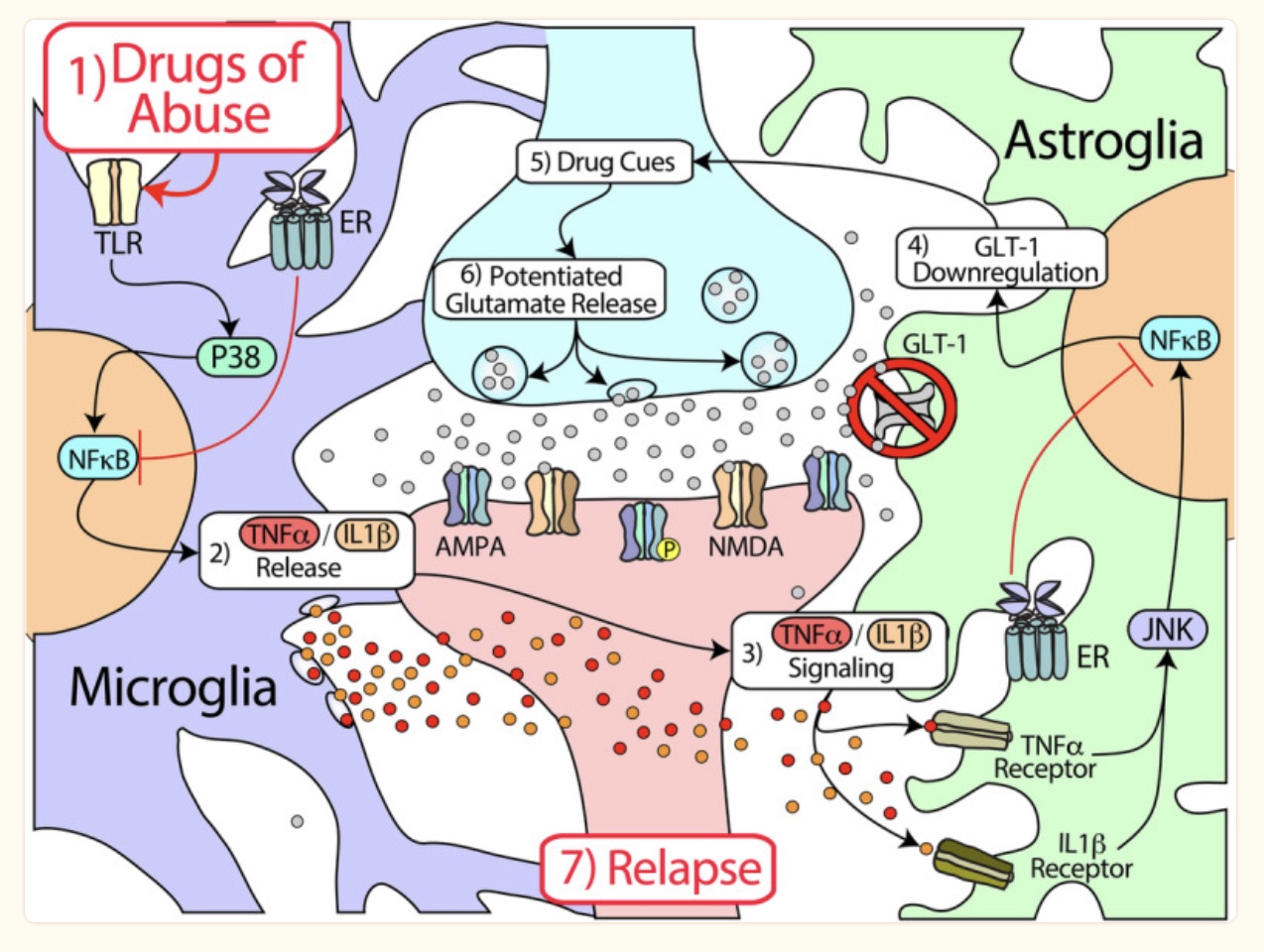
Outside the brain, glutamate can also play a role in activating the immune system. For example, glutamate can be converted to alpha-ketoglutarate for use in energy production, which makes it of interest to cancer researchers because cancer cells consume a lot of energy to grow. Glutamate receptors are also found on T cells and other immune cells, and regulation of glutamate uptake is thought to play a role in the way the immune system responds to cancer.[ref]
The role of glutamate in clotting:
While we have focused here on glutamate as a neurotransmitter, glutamate also circulates in the bloodstream.
Platelets play an essential role in forming blood clots. Platelets have glutamate receptors on their surface, and platelet-dense granules carry glutamate, which is released when activated.
A 2019 study showed that glutamate can induce the formation of clot-forming peptides, such as plasminogen activator inhibitor-1 (PAI-1). Glutamate also induced platelet adhesion, switching the platelets to “pro-activation phenotype”.[ref]
Glutamate released from activated platelets may also play a role in Alzheimer’s and Parkinson’s diseases.[ref]
I’m only scratching the surface here on the research studies on glutamate. If you’re interested in learning more, I encourage you to check out the [ref] links – there are some excellent and easily readable studies. For now, let’s look at the genetic variants that impact glutamate levels and then the lifehacks / solutions for modulating glutamate.
Genotype report:
This genotype section is divided into:
- glutamate synthesis SNPs
- glutamate transport
- GABA synthesis from glutamate
Keep in mind that the synthesis and release of glutamate are only part of the picture – the actions come from glutamate binding to receptors. An article on glutamate receptors is coming soon!
Glutamate synthesis genes:
GLS2 gene: glutaminase enzyme found in the mitochondria. It converts glutamine to glutamate plus ammonia.
Check your genetic data for rs2657879 (23andMe v5; AncestryDNA)
- G/G lower GLS2, lower glutamate levels[ref]; increased risk of complicated Staphylococcus aureus bacteremia[ref]
- A/G: typical
- A/A: typical (higher glutamine to glutamate compared to GG)
Members: Your genotype for rs2657879 is —.
Check your genetic data for rs2638315 (23andMe v4, v5; AncestryDNA):
- C/C: typical
- C/G: increased serum glutamine levels
- G/G: increased serum glutamine levels[ref]
Members: Your genotype for rs2638315 is —.
GLUL gene: encodes glutamine synthase, which is used to convert glutamate to glutamine. Not enough glutamine synthase could cause elevated glutamate levels along with a lack of glutamine.
Check your genetic data for rs10911021 (23andMe v4, v5; AncestryDNA):
- C/C: typical, most common genotype
- C/T: typical
- T/T: decreased relative risk of mortality in people with cardiovascular disease[ref], decreased risk of cardiovascular disease in type 2 diabetes[ref]
Members: Your genotype for rs10911021 is —.
Check your genetic data for rs80358215 (AncestryDNA):
- G/G: typical
- A/G: rare, glutamine deficiency[ref]
Members: Your genotype for rs80358215 is —.
GLUD1 gene: glutamate dehydrogenase 1, which converts glutamate to alpha-ketoglutarate (and also converts alpha-ketoglutarate to glutamate in the liver)
Note: AncestryDNA data isn’t guaranteed clinically accurate, so false negatives and false positives are possible.
Check your genetic data for rs121909730 (AncestryDNA):
- G/G: typical
- A/G: rare mutation linked to hyperinsulinemia hyperammonemia.[ref]
Members: Your genotype for rs121909730 is —.
Check your genetic data for rs121909731 (AncestryDNA):
- G/G: typical
- A/G: rare mutation linked to hyperinsulinemia hyperammonemia.[ref]
Members: Your genotype for rs121909731 is —.
Check your genetic data for rs797045597 (23andMe v5):
- C/C: typical
- C/T: rare mutation linked to hyperinsulinemia hyperammonemia[ref]
Members: Your genotype for rs797045597 is —.
Genes related to glutamate transport:
SLC1A1 gene: encodes the glutamate transporter that regulates glutamate levels in the synapse (EEAC1). Too much glutamate left in the synapse could cause excess activation of the glutamate receptors.
Check your genetic data for rs2228622 (23andMe v4, v5; AncestryDNA):
- A/A: increased relative risk of OCD[ref]
- A/G: increased relative risk of OCD
- G/G: typical
Members: Your genotype for rs2228622 is —.
Check your genetic data for rs301430 (23andMe v4, v5)
- C/C: higher expression, more anxiety in autism spectrum disorder; increased OCD risk[ref][ref]
- C/T: higher expression, more anxiety in autism spectrum disorder; increased OCD risk
- T/T: typical
Members: Your genotype for rs301430 is —.
SLC1A2 gene: glial affinity glutamate transporter
Check your genetic data for rs3794087 (23andMe v4, v5; AncestryDNA):
- T/T: increased relative risk of essential tremor (Caucasian, Taiwanese population groups)[ref][ref][ref]
- G/T: increased relative risk of essential tremor (Caucasian, Taiwanese populations)
- G/G: typical
Members: Your genotype for rs3794087 is —.
Check your genetic data for rs12294045 (23andMe v4, v5; AncestryDNA):
- T/T: increased relative risk of schizophrenia (Chinese population group)[ref]
- C/T: increased relative risk of schizophrenia (Chinese population)
- C/C: typical
Members: Your genotype for rs12294045 is —.
Genes related to creating GABA:
GABA is synthesized from glutamate using the GAD1 and GAD2 genes. Read the full article on GABA here. I’ve included these genes because it’s possible that altered GABA conversion could affect glutamate levels or combine with the glutamate synthesis variants for a larger impact.
Gene | RS ID | Effect Allele | Your Genotype | Notes About Effect Allele |
---|---|---|---|---|
GAD1 | rs3749034 | A | -- | Increased risk panic disorders |
GAD1 | rs1978340 | A | -- | AA only: higher GABA |
GAD1 | rs769390 | C | -- | CC only: higher GABA |
GAD1 | rs3791878 | T | -- | Less susceptibility to PTSD |
GABRA1 | rs2279020 | G | -- | Increased risk epilepsy; susceptibility to propofol |
GABRA1 | rs121434579 | A | -- | Increased risk epilepsy (rare) |
GABRG2 | rs211037 | T | -- | Increased risk of seizures |
ALDH5A1 | rs3765310 | T | -- | Reduced ALDH1; increased GHB in blood |
ALDH5A1 | rs2760118 | T | -- | Reduced ALDH1; increased risk of impaired cognitive function |
ALDH5A1 | rs62621664 | T | -- | Reduced ALDH1 |
GABRA2 | rs279858 | C | -- | increased risk of alcohol use disorder; increased stimulant response from alcohol; linked to aggression and alcohol use in people who had stressful life events as teens |
GABRG2 | rs796052504 | T | -- | Rare mutation linked to epilepsy |
GABRA2 | rs279871 | T | -- | T/T: increased risk of alcohol use disorder |
Lifehacks for glutamate:
If you are being treated for a psychiatric disorder, please consult your doctor before taking any supplements or even changing your diet. If you have questions about supplements and drug interactions, your pharmacist or doctor should be able to help.
Glutamate in foods:
Glutamate is found in many (most) packaged, processed foods that contain flavor enhancers such as MSG, hydrolyzed protein, yeast extract, and aspartame. Glutamate, or glutamic acid, is associated with the umami taste. Glutamate is also found naturally in higher levels in soy sauce, fish sauce, aged cheese, and Marmite. Lower levels of glutamate can be found in tomatoes, anchovies, walnuts, dried fruit, and peas.
Glutamine in foods:
Glutamine can be found in many foods. It is found in higher amounts in animal protein, eggs, and dairy products, but it is also abundant in vegetables and grains. [ref] Athletes may benefit from supplemental glutamine. Studies show that glutamine supplements post-workout help with fatigue and immune suppression.[ref]