Key takeaways:
~ Insulin is a hormone that regulates glucose levels in cells and throughout the body. It binds to receptors on cells, causing glucose transporters to move to the cell surface and take glucose into the cell.
~ The term insulin resistance indicates that the signal from insulin is not properly received by cells and the receptors do not move to the cell surface to take up more glucose.
~ Insulin resistance can lead to diabetes. Even before diabetes develops, insulin resistance can have negative health consequences.
~ Genetic variants can make you more or less likely to develop insulin resistance, depending on your diet.
~ Importantly, genetics can show which cellular pathways are involved, including the role of BCAAs in insulin resistance.
Members will see their genotype report below, plus additional solutions in the Lifehacks section. Consider joining today.
What is insulin resistance?
Insulin resistance is a condition in which the body’s cells don’t respond properly to insulin. This can lead to metabolic syndrome, fatty liver disease, atherosclerosis, and diabetes. Depending on the test value used to define it, insulin resistance likely affects 14-23% of the adult population.[ref]
Insulin is an endocrine hormone produced by beta cells in the pancreas. When you eat foods that contain carbohydrates, your body breaks them down into glucose for use as energy in cells. Cells can take up glucose in one of two ways: insulin-mediated glucose uptake or non-insulin-mediated glucose uptake. When you’re fasting, most of a cell’s glucose uptake is non-insulin-mediated.[ref] Our focus here for insulin resistance (IR) is the response to eating – or more specifically, eating foods that contain carbohydrates.
Insulin is released by the pancreas in response to rising glucose levels in the bloodstream. Insulin binds to receptors on the surface of cells – mainly liver, muscle, and fat cells- causing the cell to move more glucose transporters to the surface of the cell. This causes the cell to take up more glucose to balance the amount of glucose in the bloodstream.
This ability to store more glucose in muscle, fat, and the liver is how we balance glucose between meals. We don’t have to eat all the time because we can store the glucose as fat in adipose tissue or glycogen (liver and muscles) for use when we’re not eating.
What goes wrong in insulin resistance (IR)?
In insulin resistance, the liver, muscles, and fat begin to respond poorly to insulin and take up less glucose. As a result, more insulin is needed to get glucose into cells. This leads to metabolic syndrome.
Researchers don’t fully understand what goes wrong to trigger IR, but several known mechanisms are involved.
Insulin binds to the insulin receptor (IGF1, INSR gene) on the surface of cells, which causes several things to happen inside the cell:[ref]
- In muscle cells, insulin binding to the receptor causes the glucose transporter, GLUT4, to move to the surface of the cell and take up more glucose. It also causes the muscle cells to make more glycogen.
- In liver cells, insulin stops the production of more glucose, by inhibiting gluconeogenesis, and increases the production of fat for storage.
- In fat cells (adipose tissue), insulin causes GLUT 4 to move to the surface to take in more glucose. It also causes an increase in fat synthesis (lipogenesis) and a decrease in the conversion of fat to free fatty acids for use as energy (lipolysis).
Insulin resistance can be due to the liver not responding to the insulin signal as it should. Essentially, the liver should stop producing more glucose when it gets the signal from insulin that blood glucose levels are already high enough. In IR, the liver doesn’t get the full ‘stop’ signal.[ref][ref]
In skeletal muscle, insulin resistance is associated with a decrease in GLUT4 on the cell surface. Researchers think it is a lack of the GLUT4 receptor getting the signal to move to the cell surface, rather than a problem with the number or function of GLUT4.
Inside skeletal muscle cells, the signal from insulin triggers the PI3K/AKT pathway. This is one place researchers think things go wrong in insulin resistance, and the signal doesn’t trigger GLUT4 translocation.[ref]
Without enough GLUT4 receptors moving to the surface of the cell, not enough glucose is absorbed out of the bloodstream. The higher blood glucose levels then trigger the release of more insulin, sending a stronger signal to cells to take in glucose.
Interestingly, some people may have selective insulin resistance in which a specific tissue, such as the liver, doesn’t respond properly to the insulin signal.[ref]
Symptoms and Testing for Insulin Resistance:
Often, insulin resistance has no noticeable symptoms at first, but loss of glucose tolerance can eventually lead to signs such as fatigue, hunger, and high blood sugar levels.
The most common tests doctors order include fasting blood glucose or HbA1c. A high fasting glucose level indicates prediabetes or diabetes, but it doesn’t necessarily indicate the early stages of insulin resistance. HbA1c levels reflect an average of blood glucose levels over several months and are better at showing diabetes or prediabetes than insulin resistance. However, an HbA1c that is trending upward may indicate insulin resistance.
Better results for insulin resistance can be found with the following tests, which are often used in research studies:
- Homeostatic Model Assessment (HOMA-IR): This is a calculated value that includes insulin levels and blood glucose levels to determine insulin resistance.
- Hyperinsulinemic-Euglycemic Clamp: Considered the gold standard for measuring insulin sensitivity, this more invasive lab test involves infusing insulin or glucose and measuring the body’s response.
Diabetes and insulin resistance:
You may be thinking that insulin resistance sounds like diabetes… and IR is usually a component of type 2 diabetes.
While most people who have type 2 diabetes are insulin resistant, insulin resistance alone doesn’t cause diabetes.[ref] Diabetes is defined by high blood glucose levels that are not kept under control by insulin. This is often due to insulin resistance plus a decrease in insulin production.
Insulin resistance alone impacts health:
Even if you produce enough insulin to prevent type 2 diabetes, insulin resistance can have negative health consequences. For example, insulin resistance alone increases the risk of cardiovascular disease.[ref]
Underlying causes of insulin resistance:
Let’s switch gears and dive into the research studies on what causes insulin resistance. While the obvious answer is “too much sugar,” research shows that it is more complex than just eating carbohydrates.
Branched-Chain Amino Acids and Insulin Resistance:
The three branched-chain amino acids (BCAAs) are leucine, isoleucine, and valine. These amino acids are found in meat, dairy products, whole grains, nuts, and seeds – most protein-rich foods – and are essential amino acids, meaning that you must get them from food because your body can’t synthesize them.
Branched-chain amino acids can be used by the body in three ways:
- as building blocks for muscle protein
- as a signaling molecule
- for energy production in the mitochondria
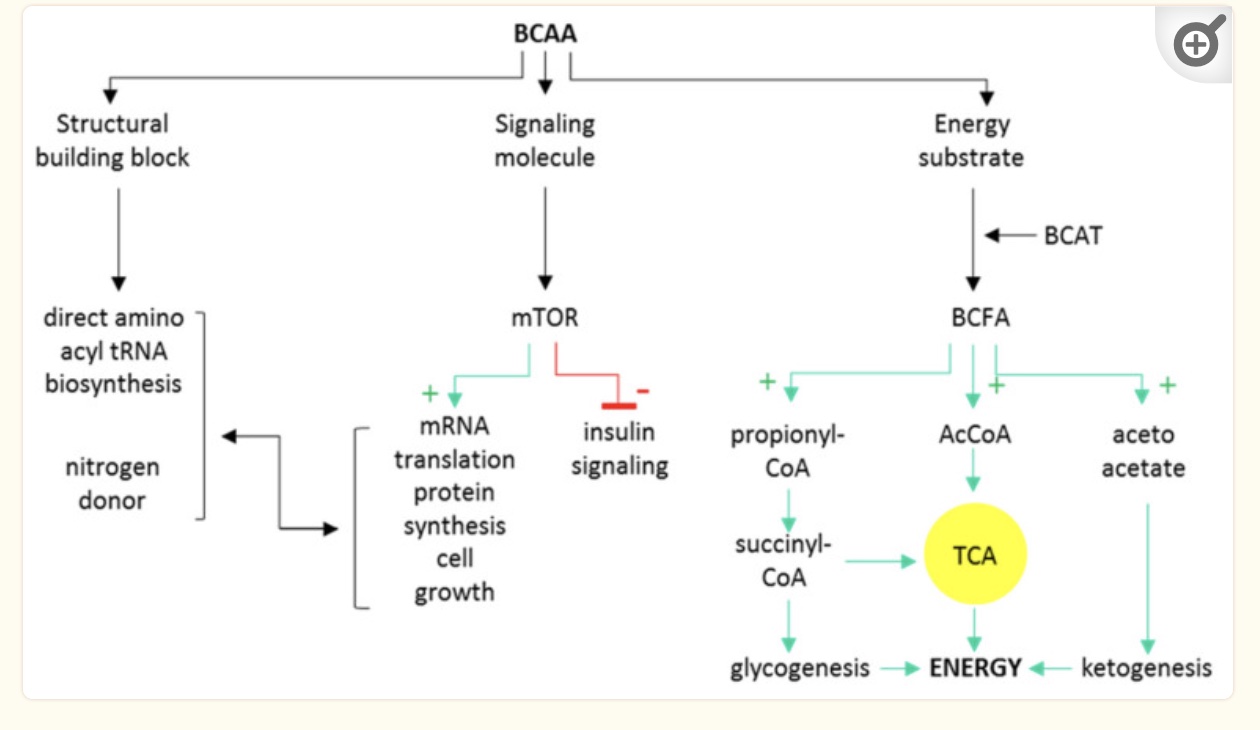
Decades of studies have repeatedly shown that people with insulin resistance have elevated BCAA levels compared to people without insulin resistance. [ref] Additionally, studies show that high BCAA levels are predictive of getting diabetes in the future.[ref][ref] Plus, studies show that even for healthy adults (across multiple ethnic groups) there is a correlation between higher BCAA levels and higher fasting insulin.[ref]
Animal studies explain why and how high BCAA levels are related to insulin resistance.
In an in-depth study, researchers fed rats three diets – a high-fat diet, a high-fat diet supplemented with BCAA, and a standard chow diet. The animals on the high-fat diet gained the most weight, and the high-fat plus BCAA diet group had about the same weight gain as the control group. However, the difference in body weight was because the BCAA group ate significantly less. While this sounds like a win — adding branched-chain amino acids decreased appetite and weight gain — the high-fat plus BCAA group became insulin resistant, just like the obese animals. This means that the contribution of branched-chain amino acids to insulin resistance is independent of obesity. The researchers then did another experiment and found that simply adding BCAA to the standard chow did not cause any changes in weight or insulin resistance. Thus, BCAAs, along with a high-fat diet, appear to be a cause of insulin resistance. One notable difference is that adding the BCAA to a high-fat diet increased mTOR activity more than a high-fat diet alone.[ref]
Is dietary fat along with BCAAs a problem in humans? A 4-week dietary intervention study showed that a high saturated fat diet plus increased protein caused both higher BCAA levels and more insulin resistance. This was compared to a lower-fat diet.[ref]
Researchers hypothesize that the standard American diet, which is high in both fat and protein (especially BCAAs), promotes insulin resistance. A relative lack of IGF-1 would then cause the BCAAs to be diverted from protein synthesis into catabolic pathways, resulting in an increase in specific BCAA metabolites.[ref] (Check your IGF-1 gene in the genotype report below)
A key question: Do BCAAs cause insulin resistance, or are elevated BCAAs a byproduct of metabolic syndrome?
Genetic research can be used to determine whether something is likely causal – in this case, whether higher BCAA levels cause insulin resistance and ultimately diabetes.
Here are three genetic studies that show likely causality for BCAA’s in IR:
- A Mendelian randomization (MR) study shows that the genetic variants that increase BCAA levels, such as PPM1K below in the genotype report, are associated with an approximately 50-85% increased relative risk of developing diabetes.[ref]
- Another Mendelian randomization study found that variants in the BCKD gene, which encodes a rate-limiting enzyme for BCAA catabolism, likely show a causal role for BCAAs in insulin resistance and type 2 diabetes.[ref]
- Yet another MR study found that genetically higher concentrations of isoleucine, leucine, and valine (the BCAAs) elevated the risk of IR.[ref]
- A study looking at genetic risk scores for diabetes found that high BCAA intake is a risk for type 2 diabetes only in people with genetic susceptibility.[ref]
One study concludes: “Taken together with existing studies, this implies that branched-chain amino acid metabolism lies on a causal pathway from adiposity and insulin resistance to type 2 diabetes.”[ref]
The next question: If higher BCAA levels cause IR, does reducing BCAA intake reverse IR?
A randomized, placebo-controlled, double-blind, crossover trial in people with diabetes looked at the effect of reducing BCAAs a little bit while increasing other amino acids to make protein levels equal. They found that reducing BCAAs a little bit resulted in increased glucose sensitivity and increased FGF21.[ref]
More evidence comes from a recent drug trial that found that accelerating BCAA breakdown caused a 27% improvement in insulin sensitivity in adults with well-controlled type 2 diabetes. [ref]
Final question: Why do high BCAA levels cause insulin resistance?
BCAAs that aren’t used to build new protein, such as to increase muscle mass, are broken down primarily in muscle for use in mitochondrial energy production. The BCAA catabolic pathway uses several enzymes, including branched-chain aminotransferase (BCAT) and branched-chain alpha-ketoacid dehydrogenase (BCKDH). The BCKDH enzyme step involves activation by PPM1K, which you will see below in the genotype report is strongly associated with insulin resistance.[ref]
The intermediates produced from the catabolism of branched-chain amino acids for energy production in the mitochondria are theorized to cause insulin resistance. Indeed, animal studies show this is true and reversible. For example, increasing the branched-chain α-keto acid dehydrogenase enzyme reduces the accumulation of BCAA and metabolites (branched-chain ketone acids, BCKAs) which then reverses IR in mice.[ref]
Animal studies also show that higher branched-chain ketone acids (BCKAs) in muscle cells directly decrease glucose uptake by inhibiting insulin-induced AKT phosphorylation, preventing GLUT4 from relocating to the cell surface.[ref]
Additionally, higher BCAAs also cause a number of changes in signaling molecules that directly affect insulin sensitivity. FGF21 increases with low BCAAs and decreases with high BCAAs. FGF21 signals to the brain and causes increased insulin sensitivity and energy expenditure. Abnormally high BCAAs would then cause low FGF21. In addition, higher levels of BCAAs are a signal to mTOR that protein is plentiful and insulin signaling should be downregulated.[ref]
Could IR be only due to eating too much fat?
Dietary sources of branched-chain amino acids, such as steak, cheese, and nuts, also tend to be high in fat, leading some researchers to wonder if it is the high-fat content alone that causes IR.
Feeding a high-fat diet to mice can cause insulin resistance, and in humans, epidemiological studies show that high fat intake is associated with insulin resistance. For example, one study showed that an increase of 40 grams of fat per day was associated with a 3.4-fold increased risk of type 2 diabetes.[ref]
However, a number of other studies have found that there is no correlation between fat intake and diabetes, although both short- and medium-chain fatty acids are potentially beneficial for insulin sensitivity.[ref] Storing excess fat in the liver or skeletal muscle is strongly associated with insulin resistance.
A ketogenic diet is very low in carbs and high in fat and BCAAs from protein. Many individuals have successfully used the keto diet to lose weight and improve their blood glucose levels. In animals, a ketogenic diet reduces body weight and increases energy expenditure. However, the hyperinsulinemic, euglycemic clamp tests show that the ketogenic diet causes insulin resistance in the liver.[ref][ref][ref][ref]
While fat intake may be involved in IR, dietary fat alone is probably not the primary cause of insulin resistance. Instead, the answer may be that high fat along with dysregulation of BCAA metabolites is the key.
Wait – what about sugar??
When I started looking into the research on insulin resistance, I expected to find that sugar consumption was the sole driver of insulin resistance. Sugar makes you fat and causes diabetes… right? While very high levels of sugar intake may play a role, it doesn’t seem to be as simple as I assumed.
Researchers tested how fructose, glucose, and saturated fat separately affect insulin sensitivity in the liver. Healthy adults were tested on a baseline diet for one week, and then the participants were divided into groups with an overfed diet containing either 1.5, 3, or 4 g/kg/day of fructose, or 3 g/kg/day of glucose, or 30% excess energy from saturated fat. The results showed that only the groups consuming 3 to 4 g/kg/day of extra fructose had a detrimental effect on liver insulin sensitivity.[ref] (For a 180 lb adult, this would be 246 g to 328 g of fructose added to their normal diet each day. For reference, a 12-ounce can of cola contains about 39 grams of fructose, and 1 cup of apple juice contains 14 grams of fructose).
While a high intake of fructose decreases insulin sensitivity, a moderate intake of 100g/day or less seems to have little effect on insulin sensitivity, at least in healthy adults. Instead, some researchers theorize that sugar just means too many calories: “Dietary sugar overconsumption promotes a positive energy balance, thereby increasing body weight and fat deposition and indirectly leading to insulin resistance and glucose dysregulation.”[ref] Just as a note – sugar consumption and sugar-sweetened beverage consumption peaked in the mid-90s and has been declining for a couple of decades.[ref]
So we have an excess of BCAAs that is strongly linked to causing insulin resistance, with fat and fructose probably playing some role as well. The idea that there may be multiple causes of insulin resistance makes sense, and perhaps genetics can help shed more light here on what is important for an individual…
Which genes play a role in insulin resistance?
Every nutrition study out there seems to include assumptions made by the researchers designing the study (e.g., vegan vs. keto, sugar vs. saturated fat).
To figure out the true cause of a condition, researchers often turn to genetic studies to sort out which pathways are involved without the inherent cognitive biases.
The heritability of insulin resistance is estimated by researchers to be 40-50% after adjusting for BMI, waist circumference, etc.[ref]
This means that genetics plays a significant role in whether someone will have insulin resistance, but diet and lifestyle are also very important.
genetics + environment (food, exercise, stress) = insulin resistance
The genetic component of IR explains why some people can eat junk food and drink soda without developing insulin resistance — and it also explains why the rest of us need to eat healthier and avoid high-fructose drinks.
Genome-wide association studies (GWAS) are used to determine which genetic variants increase the risk of a trait or disease. As mentioned above, the PMM1K gene, which is involved in BCAA catabolism, has been linked to insulin resistance in a number of genetic studies, including a GWAS.[ref]
A large GWAS study in 2015 found that a genetic variant in the N-acetyltransferase 2 (NAT2) gene was linked to insulin resistance. NAT2 is the rate-limiting step in multiple acetylation pathways. A mouse study that used mice with the NAT2 gene knocked down backed up the results, showing that this acetylation pathway is involved in insulin resistance.[ref] Further animal studies replicated the NAT2 GWAS finding.
Acetylation can affect the amount of proteins produced, including GLUT4, Akt, IGF-1 receptor, and other proteins directly involved in insulin signaling.[ref] The NAT2 gene also ties into detoxification and chronic inflammation.
Note: Many IR genetic variants have been identified, most of which involve type 2 diabetes along with insulin resistance. While it can be difficult to disentangle the genes involved in diabetes from those involved in insulin resistance, the genotype report below focuses narrowly on insulin resistance and the pathways involved. For the other half of the diabetes picture (reduced insulin production, circadian rhythm, and other diabetes risk factors), please see the Type 2 Diabetes Genetic Risk report.
Genotype report: Insulin resistance
BCAA metabolism and amino acid sensing:
PPM1K gene: mitochondrial gene involved in BCAA metabolism, it activates one of the mitochondrial branched-chain alpha-ketoacid dehydrogenase enzymes responsible for the rate-limiting step in BCAA catabolism.
Check your genetic data for rs1440581 (23andMe v4, v5; AncestryDNA):
- C/C: On average, higher BCAA levels compared to TT. For people on a reduced-calorie diet, a low-fat diet worked better for reducing insulin resistance.[ref][ref] (common genotype)
- C/T: Both the low-fat and high-fat diet groups had similar reductions in IR and intermediate BCAA levels.
- T/T: On average, lower BCAA and lower cardiovascular disease risk (good!)[ref]A high-fat, reduced-calorie diet worked better for decreasing insulin resistance.[ref]
Members: Your genotype for rs1440581 is —.
Check your genetic data for rs9637599 (23andMe v4; AncestryDNA):
- A/A: typical
- A/C: On average, higher BCAA levels[ref] Increased risk of insulin resistance and diabetes with high BCAA intake.[ref]
- C/C: On average, higher BCAA levels[ref] Increased risk of insulin resistance and diabetes with high BCAA intake.[ref]
Members: Your genotype for rs9637599 is —.
BCAT1 gene: encodes the enzyme branched-chain amino acid transaminase 1
Check your genetic data for rs2242400 (23andMe v4; AncestryDNA):
- A/A: typical
- A/G: increased BCAAs, increased risk of diabetes
- G/G: increased BCAAs, increased risk of diabetes[ref][ref]
Members: Your genotype for rs2242400 is —.
GPRC6A gene: encodes a receptor for amino acids, osteocalcin, and testosterone. Activation of this receptor in the pancreas stimulates insulin release.[ref]
Check your genetic data for rs2274911 (23andMe v4, v5)
- A/A: most common genotype
- A/G: typical risk
- G/G: decreased risk of insulin resistance ref]
Members: Your genotype for rs2274911 is —.
Insulin signaling and gluconeogenesis:
IRS1 gene (receiving the insulin signal): Genetic variants in this gene have associations with insulin resistance and hyperinsulinemia.[ref]
Check your genetic data for rs1801278 G972R (23andMe v4):
- C/C: typical
- C/T: impaired IRS1 signaling, increased risk of insulin resistance and diabetes
- T/T: impaired IRS1 signaling,[ref][ref], increased risk of insulin resistance and diabetes[ref][ref] (rare genotype)
Members: Your genotype for rs1801278 is —.
Check your genetic data for rs2943641 (23andMe v4, v5; AncestryDNA)
- C/C: most common genotype, but a higher risk for diabetes compared to T/T[ref], lower fasting glucose levels in people without diabetes[ref] increased risk of insulin resistance[ref]
- C/T: typical risk
- T/T: lower risk of type 2 diabetes in people with high vitamin D levels[ref]
Members: Your genotype for rs2943641 is —.
ENPP1 gene (receiving the insulin signal): interacts with the insulin receptor and causes the GLUT4 receptor to move to the cell surface and take in insulin
Check your genetic data for rs1044498 (23andMe v4; AncestryDNA):
- C/C: increased risk of insulin resistance, metabolic syndrome[ref]
- A/C: increased risk of insulin resistance, metabolic syndrome
- A/A: typical
Members: Your genotype for rs1044498 is —.
PCK1 gene: encodes the cytosolic enzyme involved in the regulation of gluconeogenesis
Check your genetic data for rs2179706 (23andMe v4; AncestryDNA):
- C/C: When consuming higher omega-3 PUFA, individuals had lower insulin resistance levels on average[ref]
- C/T: Omega-3 levels didn’t affect insulin resistance
- T/T: Omega-3 levels didn’t affect insulin resistance.
Members: Your genotype for rs2179706 is —.
IGF1 gene: insulin-like growth factor 1. IGF-1 is primarily known for its role as a growth factor that promotes normal muscle and bone growth, but it can also bind to insulin receptors and stimulate glucose transport[ref]
Check your genetic data for rs35767 (AncestryDNA):
- G/G: lower insulin sensitivity, increased risk of insulin resistance[ref][ref]
- A/G: increased risk of insulin resistance
- A/A: typical
Members: Your genotype for rs35767 is —.
Modifying gene expression:
NAT2 gene: encodes an enzyme that is involved in the detoxification of certain drugs and carcinogens. It works by acetylating – adding an acetyl group – to other proteins.
Check your genetic data for rs1208 A803G (23andMe v4, v5; AncestryDNA):
- A/A: typical genotype, higher risk of insulin resistance[ref]
- A/G: possibly slower NAT2; decreased risk of insulin resistance
- G/G: likely slower NAT2[ref] decreased risk of insulin resistance
Members: Your genotype for rs1208 is —.
Lifehacks:
To reduce insulin resistance, the experts sometimes seem to recommend opposite diets, which can make it hard to know what is right.
Here are a few examples:
- Nebraska Medicine recommends whole wheat bread, whole grain pasta, lean red meat, chicken, Greek yogurt, and lentils.[ref] (High carb, moderate protein, low fat.)
- Virta Health is reversing type 2 diabetes with a ketogenic diet. (High fat, high protein, very low carb)[ref]
- Others point to vegan diets for reversing insulin resistance.[ref] (High carb, moderate fat, low protein)
A large, year-long study looked at the difference in insulin secretion and weight loss for a high-fat, low-carbohydrate diet versus a low-fat, high-carbohydrate diet. The results showed that, on average, there were no differences between the diets, although some individuals lost more (or gained weight) on each diet.[ref]
I don’t have a one-size-fits-all answer here. Instead, I suggest that you look at your genetic variants and see how the following suggestions might apply to you. Talk to your doctor if you need medical advice or a prescription.
Exercise: why and how much?
In insulin resistance, the movement of GLUT4 to the cell surface in response to insulin is impaired. However, exercise also causes GLUT4 receptors to move to the cell membrane (independent of insulin). Thus, exercise helps insulin resistance and diabetes by stimulating glucose uptake in an insulin-independent manner. Studies involving people with diabetes show that exercise lowers blood sugar levels and A1c levels (a little).[ref]
ENPPI variant: The good news here is that a randomized controlled trial shows lifestyle intervention — exercising and weight loss — completely mitigated the increased risk from this variant.[ref] This ENPP1 variant essentially downregulates the signal from insulin to GLUT4 (which brings the glucose into the cell). Exercise is a way around this.
Decreasing BCAAs:
People with any of the BCAA-related variants may want to consider reducing their intake of branched-chain amino acids. Foods highest in BCAAs include (in order) poultry, fish, eggs, beef, pork, beans, and wheat. Higher BCAA intake is strongly linked to insulin resistance in people with genetic susceptibility.[ref]
Here’s a breakdown of our ‘best’ sources of BCAAs in the diet:[ref]
Food | Serving size | BCAA content |
Whey protein powder | 1 scoop (25 g) | 5.9 g |
Chicken breast | 3.5 oz (100 g) | 5.5 g |
Canned tuna | 3.5 oz (100 g) | 4.6 g |
Ground beef, 90% lean | 3.5 ounces (oz) (100 g) | 4.5 g |
Pea protein powder | 1 scoop (25 g) | 4.5 g |
Greek yogurt | 1 cup (227 g) | 4.1 g |
Salmon | 3.5 oz (100 g) | 4 g |
Ground turkey, 93% lean | 3.5 oz (100 g) | 3.2 g |
Eggs | 2 eggs | 2.6 g |
Milk, 1% | 8 oz (237 milliliters) | 1.7 g |
Insulin resistance and type 2 diabetes rates have risen over the last 50 years. And the trend doesn’t exactly match with sugar intake, which peaked in the 90s. So I decided to look at whether we as a society are just eating more protein. Instead, I found an interesting visualization on the flowing data website that shows total protein being relatively stable, but that our protein sources have changed.
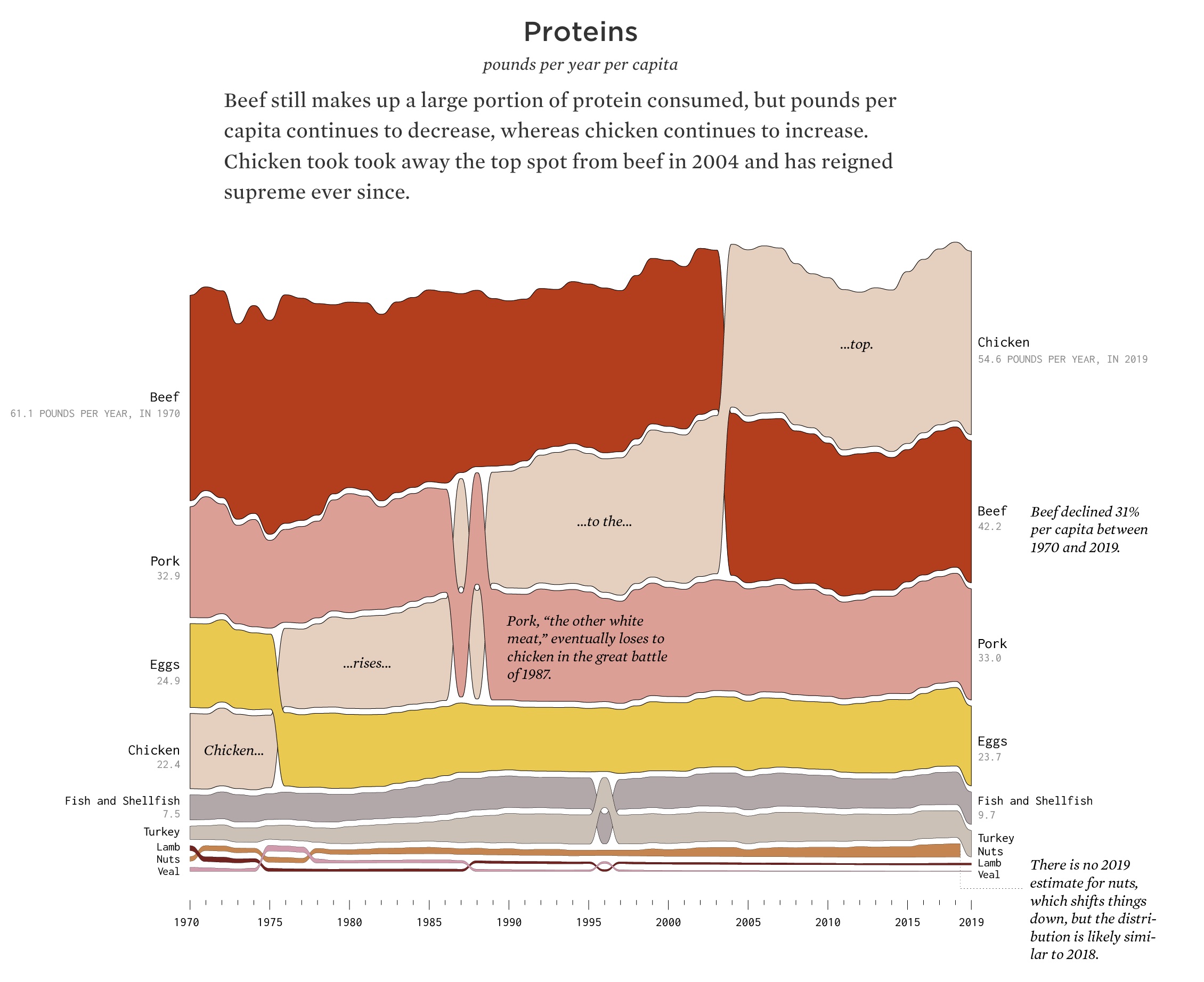
Chicken, which averages 1g more BCAAs per serving than beef, has gone from a small portion of our dietary protein in 1970 to the largest source of dietary meat. Yogurt has also grown tremendously in popularity, from a small portion of our dairy consumption in 1970 to an average of more than 9 pounds per year today. Are these changes enough to show that we are eating more BCAAs and therefore have more insulin resistance? I don’t know. Maybe it is glyphosate (or some other environmental toxin) along with an increase in BCAAs in the diet.[ref]
I don’t want anyone to take this as a blanket statement that reducing animal protein or cutting out chicken is the solution for everyone with insulin resistance — protein in the diet is important, and there’s probably a more nuanced answer here. For example, age and fitness levels matter. BCAAs are essential for building muscle, especially if you are young and/or lift weights. However, I would recommend that you read the research on BCAAs and insulin resistance, taking into account your diet, age, and activity level.
Plus, dietary BCAAs may not be the whole picture…
You are what you eat — along with what your gut microbiome produces.
Probiotics, BCAAs, and fiber:
The gut microbiome is linked to health in many ways, including influencing insulin resistance. Animal studies and clinical trials point to the positive benefits of several species of Lactobacillus and Bifidobacterium for IR.[ref]
Interestingly, the gut microbiome is also a source of amino acids. Some bacteria use dietary fiber to produce amino acids, and branched-chain amino acids (BCAAs) are abundantly produced by certain strains of gut bacteria.[ref]
A 2016 study in Nature explained that individuals with insulin resistance had higher gut microbial production of BCAAs. Two gut microbe species, Prevotella copri and Bacteroides vulgatus, were identified as the main drivers of the association between gut BCAA production and insulin resistance. The researchers then created a mouse model that showed that simply giving the mice Prevotella copri was enough to cause insulin resistance and higher levels of BCAA.[ref]
Akkermansia muciniphila has been studied extensively after low levels of the bacteria were linked to obesity and diabetes. Supplementation with A. muciniphila as a probiotic has been shown to improve insulin sensitivity in a small placebo-controlled trial.[ref]
A placebo-controlled study in women with both insulin resistance and gestational diabetes showed that a probiotic supplement containing Bifidobacterium and Lactobacillus species statistically improved insulin sensitivity.[ref]
Finally, I wanted to mention that some gut bacteria directly take in BCAAs in the intestines. Higher levels of Faecalibacterium prausnitzii cause a decrease in circulating BCAA levels and this is associated with lower levels of insulin resistance.[ref] While Faecalibacterium prausnitzii is not available as a probiotic, increasing inulin in the diet helps to promote it. Additionally, pomegranate extract and resveratrol may also promote it.[ref]
Increasing glycine:
In addition to BCAA being higher than normal in people with insulin resistance, another amino acid, glycine, is lower than normal.[ref] Low levels of glycine are also predictive of future type 2 diabetes.
Mechanistically, there is a feedback loop with glycine receptors on the beta islet cells that release insulin in the pancreas. Animal studies show that glycine, along with sugar, reduces the effects of insulin resistance. In addition, glycine can affect glutathione levels, and glutathione acts as an antioxidant and reduces damage from oxidative stress[ref]
Glycine is abundant in bone broth, gelatin, and collagen. Historically, people used to consume much more glycine, both in the form of broths and by consuming the whole animal.
Decreasing sugar and fructose:
The glucose-related genetic variants are associated with a detrimental effect of overconsumption of table sugar and fructose in the diet. Reducing the amount of sugar or processed carbohydrates in the diet directly leads to lower postprandial blood glucose levels. This may be even more important for people with IRS1 variants, as IRS1 interacts with fructose and liver fat.[ref][ref]
A ketogenic diet is an obvious solution for high blood glucose levels, and this type of very low carbohydrate diet effectively lowers glucose levels and A1c numbers. However, animal studies show that a keto diet worsens insulin resistance in the liver. So a lot of questions remain as to whether a long-term keto diet is healthy for the liver and IR. In people with diabetes, though, there seems to be a clear advantage to a very low-carb diet.[ref][ref] It may very well be that the benefits of weight loss and lowering blood glucose levels with keto outweigh any possible problems with keto and liver fat. I would suggest getting yearly lab work done to make sure all is good.
Bringing this together with multiple changes
A picture emerges from the research of multiple pathways to reverse or prevent insulin resistance.
- Cutting down on foods high in BCAAs may also help to reduce the excess BCAA levels that cause IR.
- Focusing on the gut microbiome seems to be a key element, but it is also hard to know what is actually going on there.
- Reducing sugar, if you eat too much of it, still seems like the most logical way to reduce high blood sugar and the need for insulin.
- Consider increasing glycine in your diet if it is low.
I would suggest tracking your normal diet for a few days or a week using cronometer.com, a free website that lets you track what you eat and see the breakdown of macronutrients and amino acids. Look at how much isoleucine, leucine, and valine you normally consume and identify which food sources of these BCAAs you consume in abundance. Then look at both sugar and glycine to see if you need to make changes there. Talk with your doctor or a dietician if you need help.
Natural Supplements for IR:
Related articles:
Targeting the GLP-1 Receptor: Genetics and a New Option for Weight Loss
References:
“5 Proven Benefits of BCAAs (Branched-Chain Amino Acids).” Healthline, 11 July 2018, https://www.healthline.com/nutrition/benefits-of-bcaa.
Almind, K., et al. “A Common Amino Acid Polymorphism in Insulin Receptor Substrate-1 Causes Impaired Insulin Signaling. Evidence from Transfection Studies.” The Journal of Clinical Investigation, vol. 97, no. 11, June 1996, pp. 2569–75. PubMed, https://doi.org/10.1172/JCI118705.
Banaszak, Michalina, et al. “Non-Pharmacological Treatments for Insulin Resistance: Effective Intervention of Plant-Based Diets—A Critical Review.” Nutrients, vol. 14, no. 7, Mar. 2022, p. 1400. PubMed Central, https://doi.org/10.3390/nu14071400.
—. “Non-Pharmacological Treatments for Insulin Resistance: Effective Intervention of Plant-Based Diets—A Critical Review.” Nutrients, vol. 14, no. 7, Mar. 2022, p. 1400. PubMed Central, https://doi.org/10.3390/nu14071400.
—. “Non-Pharmacological Treatments for Insulin Resistance: Effective Intervention of Plant-Based Diets—A Critical Review.” Nutrients, vol. 14, no. 7, Mar. 2022, p. 1400. PubMed Central, https://doi.org/10.3390/nu14071400.
Bielohuby, Maximilian, et al. “Impaired Glucose Tolerance in Rats Fed Low-Carbohydrate, High-Fat Diets.” American Journal of Physiology. Endocrinology and Metabolism, vol. 305, no. 9, Nov. 2013, pp. E1059-1070. PubMed, https://doi.org/10.1152/ajpendo.00208.2013.
Biswas, Dipsikha, et al. “Branched-Chain Ketoacid Overload Inhibits Insulin Action in the Muscle.” The Journal of Biological Chemistry, vol. 295, no. 46, Nov. 2020, pp. 15597–621. PubMed, https://doi.org/10.1074/jbc.RA120.013121.
Depommier, Clara, et al. “Supplementation with Akkermansia Muciniphila in Overweight and Obese Human Volunteers: A Proof-of-Concept Exploratory Study.” Nature Medicine, vol. 25, no. 7, July 2019, pp. 1096–103. PubMed, https://doi.org/10.1038/s41591-019-0495-2.
Di Nisio, Andrea, et al. “The Rs2274911 Polymorphism in GPRC 6A Gene Is Associated with Insulin Resistance in Normal Weight and Obese Subjects.” Clinical Endocrinology, vol. 86, no. 2, Feb. 2017, pp. 185–91. DOI.org (Crossref), https://doi.org/10.1111/cen.13248.
Ding, Yilan, et al. “Unlocking the Potential: Amino Acids’ Role in Predicting and Exploring Therapeutic Avenues for Type 2 Diabetes Mellitus.” Metabolites, vol. 13, no. 9, Sept. 2023, p. 1017. PubMed Central, https://doi.org/10.3390/metabo13091017.
Dupuis, Josée, et al. “New Genetic Loci Implicated in Fasting Glucose Homeostasis and Their Impact on Type 2 Diabetes Risk.” Nature Genetics, vol. 42, no. 2, Feb. 2010, pp. 105–16. PubMed Central, https://doi.org/10.1038/ng.520.
Ellenbroek, Johanne H., et al. “Long-Term Ketogenic Diet Causes Glucose Intolerance and Reduced β- and α-Cell Mass but No Weight Loss in Mice.” American Journal of Physiology. Endocrinology and Metabolism, vol. 306, no. 5, Mar. 2014, pp. E552-558. PubMed, https://doi.org/10.1152/ajpendo.00453.2013.
Emamgholipour, Solaleh, et al. “Acetylation and Insulin Resistance: A Focus on Metabolic and Mitogenic Cascades of Insulin Signaling.” Critical Reviews in Clinical Laboratory Sciences, vol. 57, no. 3, Jan. 2020, pp. 196–214. PubMed, https://doi.org/10.1080/10408363.2019.1699498.
Gardner, Christopher D., et al. “Effect of Low-Fat vs Low-Carbohydrate Diet on 12-Month Weight Loss in Overweight Adults and the Association With Genotype Pattern or Insulin Secretion.” JAMA, vol. 319, no. 7, Feb. 2018, pp. 667–79. PubMed Central, https://doi.org/10.1001/jama.2018.0245.
Gilsing, Anne M. J., et al. “Meat-Related Mutagen Exposure, Xenobiotic Metabolizing Gene Polymorphisms and the Risk of Advanced Colorectal Adenoma and Cancer.” Carcinogenesis, vol. 33, no. 7, July 2012, pp. 1332–39. PubMed, https://doi.org/10.1093/carcin/bgs158.
Gojda, Jan, and Monika Cahova. “Gut Microbiota as the Link between Elevated BCAA Serum Levels and Insulin Resistance.” Biomolecules, vol. 11, no. 10, Sept. 2021, p. 1414. PubMed Central, https://doi.org/10.3390/biom11101414.
Goni, Leticia, et al. “Effect of the Interaction between Diet Composition and the PPM1K Genetic Variant on Insulin Resistance and β Cell Function Markers during Weight Loss: Results from the Nutrient Gene Interactions in Human Obesity: Implications for Dietary Guidelines (NUGENOB) Randomized Trial.” The American Journal of Clinical Nutrition, vol. 106, no. 3, Mar. 2017, pp. 902–08. ScienceDirect, https://doi.org/10.3945/ajcn.117.156281.
GWAS Catalog. https://www.ebi.ac.uk/gwas/variants/rs1440581. Accessed 14 Dec. 2023.
—. https://www.ebi.ac.uk/gwas/variants/rs9637599. Accessed 14 Dec. 2023.
Hatting, Maximilian, et al. “Insulin Regulation of Gluconeogenesis.” Annals of the New York Academy of Sciences, vol. 1411, no. 1, Jan. 2018, pp. 21–35. PubMed Central, https://doi.org/10.1111/nyas.13435.
Hu, Wen, et al. “Effects of PPM1K Rs1440581 and Rs7678928 on Serum Branched-Chain Amino Acid Levels and Risk of Cardiovascular Disease.” Annals of Medicine, vol. 53, no. 1, Jan. 2021, pp. 1317–27. DOI.org (Crossref), https://doi.org/10.1080/07853890.2021.1965204.
Jornayvaz, François R., et al. “A High-Fat, Ketogenic Diet Causes Hepatic Insulin Resistance in Mice, despite Increasing Energy Expenditure and Preventing Weight Gain.” American Journal of Physiology. Endocrinology and Metabolism, vol. 299, no. 5, Nov. 2010, pp. E808-815. PubMed, https://doi.org/10.1152/ajpendo.00361.2010.
Jumpertz, Reiner, et al. “Assessment of Non-Insulin Mediated Glucose Uptake: Association with Body Fat and Glycemic Status.” Metabolism: Clinical and Experimental, vol. 59, no. 10, Oct. 2010, pp. 1396–401. PubMed Central, https://doi.org/10.1016/j.metabol.2010.01.006.
Karusheva, Yanislava, et al. “Short-Term Dietary Reduction of Branched-Chain Amino Acids Reduces Meal-Induced Insulin Secretion and Modifies Microbiome Composition in Type 2 Diabetes: A Randomized Controlled Crossover Trial.” The American Journal of Clinical Nutrition, vol. 110, no. 5, Nov. 2019, pp. 1098–107. ScienceDirect, https://doi.org/10.1093/ajcn/nqz191.
Kijmanawat, Athasit, et al. “Effects of Probiotic Supplements on Insulin Resistance in Gestational Diabetes Mellitus: A Double-Blind Randomized Controlled Trial.” Journal of Diabetes Investigation, vol. 10, no. 1, Jan. 2019, pp. 163–70. PubMed, https://doi.org/10.1111/jdi.12863.
Knowles, Joshua W., et al. “Identification and Validation of N-Acetyltransferase 2 as an Insulin Gene.” The Journal of Clinical Investigation, vol. 125, no. 4, Apr. 2015, pp. 1739–51. PubMed Central, https://doi.org/10.1172/JCI74692.
Lecoultre, V., et al. “Effects of Fructose and Glucose Overfeeding on Hepatic Insulin Sensitivity and Intrahepatic Lipids in Healthy Humans.” Obesity (Silver Spring, Md.), vol. 21, no. 4, Apr. 2013, pp. 782–85. PubMed, https://doi.org/10.1002/oby.20377.
Lee, Shin-Hae, et al. “Insulin Resistance: From Mechanisms to Therapeutic Strategies.” Diabetes & Metabolism Journal, vol. 46, no. 1, Jan. 2022, pp. 15–37. PubMed Central, https://doi.org/10.4093/dmj.2021.0280.
Li, Qiuyan, et al. “Associations between Two Single-Nucleotide Polymorphisms (Rs1801278 and Rs2943641) of Insulin Receptor Substrate 1 Gene and Type 2 Diabetes Susceptibility: A Meta-Analysis.” Endocrine, vol. 51, no. 1, Jan. 2016, pp. 52–62. Springer Link, https://doi.org/10.1007/s12020-015-0770-z.
Li, Xiaozhong, et al. “Prevalence Estimates of the Insulin Resistance and Associated Prevalence of Heart Failure among United Status Adults.” BMC Cardiovascular Disorders, vol. 23, June 2023, p. 294. PubMed Central, https://doi.org/10.1186/s12872-023-03294-9.
Lotta, Luca A., et al. “Genetic Predisposition to an Impaired Metabolism of the Branched-Chain Amino Acids and Risk of Type 2 Diabetes: A Mendelian Randomisation Analysis.” PLOS Medicine, vol. 13, no. 11, Nov. 2016, p. e1002179. PLoS Journals, https://doi.org/10.1371/journal.pmed.1002179.
—. “Genetic Predisposition to an Impaired Metabolism of the Branched-Chain Amino Acids and Risk of Type 2 Diabetes: A Mendelian Randomisation Analysis.” PLOS Medicine, vol. 13, no. 11, Nov. 2016, p. e1002179. PLoS Journals, https://doi.org/10.1371/journal.pmed.1002179.
Mahmutovic, Lejla, et al. “Association of IRS1 Genetic Variants with Glucose Control and Insulin Resistance in Type 2 Diabetic Patients from Bosnia and Herzegovina.” Drug Metabolism and Personalized Therapy, vol. 34, no. 1, Mar. 2019. PubMed, https://doi.org/10.1515/dmpt-2018-0031.
Mannino, Gaia Chiara, et al. “A Fasting Insulin–Raising Allele at IGF1 Locus Is Associated with Circulating Levels of IGF-1 and Insulin Sensitivity.” PLOS ONE, vol. 8, no. 12, Dec. 2013, p. e85483. PLoS Journals, https://doi.org/10.1371/journal.pone.0085483.
Marriott, Bernadette P., et al. “Trends in Intake of Energy and Total Sugar from Sugar-Sweetened Beverages in the United States among Children and Adults, NHANES 2003–2016.” Nutrients, vol. 11, no. 9, Sept. 2019, p. 2004. www.mdpi.com, https://doi.org/10.3390/nu11092004.
Marshall, J. A., et al. “Dietary Fat Predicts Conversion from Impaired Glucose Tolerance to NIDDM. The San Luis Valley Diabetes Study.” Diabetes Care, vol. 17, no. 1, Jan. 1994, pp. 50–56. PubMed, https://doi.org/10.2337/diacare.17.1.50.
Moore, Allan F., et al. “The Association of ENPP1 K121Q with Diabetes Incidence Is Abolished by Lifestyle Modification in the Diabetes Prevention Program.” The Journal of Clinical Endocrinology and Metabolism, vol. 94, no. 2, Feb. 2009, pp. 449–55. PubMed Central, https://doi.org/10.1210/jc.2008-1583.
Mu, Liangshan, et al. “PPM1K-Regulated Impaired Catabolism of Branched-Chain Amino Acids Orchestrates Polycystic Ovary Syndrome.” eBioMedicine, vol. 89, Feb. 2023, p. 104492. PubMed Central, https://doi.org/10.1016/j.ebiom.2023.104492.
Muyyarikkandy, Muhammed S., et al. “Branched Chain Amino Acids and Carbohydrate Restriction Exacerbate Ketogenesis and Hepatic Mitochondrial Oxidative Dysfunction during NAFLD.” FASEB Journal : Official Publication of the Federation of American Societies for Experimental Biology, vol. 34, no. 11, Nov. 2020, pp. 14832–49. PubMed Central, https://doi.org/10.1096/fj.202001495R.
—. “Branched Chain Amino Acids and Carbohydrate Restriction Exacerbate Ketogenesis and Hepatic Mitochondrial Oxidative Dysfunction during NAFLD.” FASEB Journal : Official Publication of the Federation of American Societies for Experimental Biology, vol. 34, no. 11, Nov. 2020, pp. 14832–49. PubMed Central, https://doi.org/10.1096/fj.202001495R.
Newgard, Christopher B., et al. “A Branched-Chain Amino Acid-Related Metabolic Signature That Differentiates Obese and Lean Humans and Contributes to Insulin Resistance.” Cell Metabolism, vol. 9, no. 4, Apr. 2009, pp. 311–26. PubMed Central, https://doi.org/10.1016/j.cmet.2009.02.002.
Pedersen, Helle Krogh, et al. “Human Gut Microbes Impact Host Serum Metabolome and Insulin Sensitivity.” Nature, vol. 535, no. 7612, July 2016, pp. 376–81. PubMed, https://doi.org/10.1038/nature18646.
Perez-Martinez, Pablo, et al. “Gene–Nutrient Interactions on the Phosphoenolpyruvate Carboxykinase Influence Insulin Sensitivity in Metabolic Syndrome Subjects.” Clinical Nutrition, vol. 32, no. 4, Aug. 2013, pp. 630–35. ScienceDirect, https://doi.org/10.1016/j.clnu.2012.10.003.
Petersen, Max C., and Gerald I. Shulman. “Mechanisms of Insulin Action and Insulin Resistance.” Physiological Reviews, vol. 98, no. 4, Oct. 2018, pp. 2133–223. PubMed Central, https://doi.org/10.1152/physrev.00063.2017.
Salles, Bárbara Izabel Moraes, et al. “Probiotics Supplementation and Insulin Resistance: A Systematic Review.” Diabetology & Metabolic Syndrome, vol. 12, Nov. 2020, p. 98. PubMed Central, https://doi.org/10.1186/s13098-020-00603-6.
Sampath Kumar, A., et al. “Exercise and Insulin Resistance in Type 2 Diabetes Mellitus: A Systematic Review and Meta-Analysis.” Annals of Physical and Rehabilitation Medicine, vol. 62, no. 2, Mar. 2019, pp. 98–103. PubMed, https://doi.org/10.1016/j.rehab.2018.11.001.
Schugar, Rebecca C., and Peter A. Crawford. “Low-Carbohydrate Ketogenic Diets, Glucose Homeostasis, and Nonalcoholic Fatty Liver Disease.” Current Opinion in Clinical Nutrition and Metabolic Care, vol. 15, no. 4, July 2012, pp. 374–80. PubMed, https://doi.org/10.1097/MCO.0b013e3283547157.
Sesti, G., et al. “Defects of the Insulin Receptor Substrate (IRS) System in Human Metabolic Disorders.” FASEB Journal: Official Publication of the Federation of American Societies for Experimental Biology, vol. 15, no. 12, Oct. 2001, pp. 2099–111. PubMed, https://doi.org/10.1096/fj.01-0009rev.
Trautman, Michaela E., et al. “Protein Restriction and Branched‐chain Amino Acid Restriction Promote Geroprotective Shifts in Metabolism.” Aging Cell, vol. 21, no. 6, June 2022, p. e13626. DOI.org (Crossref), https://doi.org/10.1111/acel.13626.
Vanweert, Froukje, et al. “A Randomized Placebo-Controlled Clinical Trial for Pharmacological Activation of BCAA Catabolism in Patients with Type 2 Diabetes.” Nature Communications, vol. 13, June 2022, p. 3508. PubMed Central, https://doi.org/10.1038/s41467-022-31249-9.
Wang, Qin, et al. “Genetic Support for a Causal Role of Insulin Resistance on
Circulating Branched-Chain Amino Acids and Inflammation.” Diabetes Care, vol. 40, no. 12, Dec. 2017, pp. 1779–86. PubMed Central, https://doi.org/10.2337/dc17-1642.
Wang, Weiqi, et al. “Genetic Predisposition to Impaired Metabolism of the Branched Chain Amino Acids, Dietary Intakes, and Risk of Type 2 Diabetes.” Genes & Nutrition, vol. 16, no. 1, Nov. 2021, p. 20. PubMed, https://doi.org/10.1186/s12263-021-00695-3.
—. “Genetic Predisposition to Impaired Metabolism of the Branched Chain Amino Acids, Dietary Intakes, and Risk of Type 2 Diabetes.” Genes & Nutrition, vol. 16, Nov. 2021, p. 20. PubMed Central, https://doi.org/10.1186/s12263-021-00695-3.
—. “Genetic Predisposition to Impaired Metabolism of the Branched Chain Amino Acids, Dietary Intakes, and Risk of Type 2 Diabetes.” Genes & Nutrition, vol. 16, Nov. 2021, p. 20. PubMed Central, https://doi.org/10.1186/s12263-021-00695-3.
Yang, Wanbao, et al. “Regulation of Macronutrients in Insulin Resistance and Glucose Homeostasis during Type 2 Diabetes Mellitus.” Nutrients, vol. 15, no. 21, Nov. 2023, p. 4671. PubMed Central, https://doi.org/10.3390/nu15214671.
Yau, Nathan. “Seeing How Much We Ate Over the Years.” FlowingData, 8 June 2021, https://flowingdata.com/2021/06/08/seeing-how-much-we-ate-over-the-years/.
Zheng, Ju-Sheng, et al. “Circulating 25-Hydroxyvitamin D, IRS1 Variant Rs2943641, and Insulin Resistance: Replication of a Gene-Nutrient Interaction in 4 Populations of Different Ancestries.” Clinical Chemistry, vol. 60, no. 1, Jan. 2014, pp. 186–96. PubMed, https://doi.org/10.1373/clinchem.2013.215251.
Zhou, Meiyi, et al. “Targeting BCAA Catabolism to Treat Obesity-Associated Insulin Resistance.” Diabetes, vol. 68, no. 9, Sept. 2019, pp. 1730–46. PubMed Central, https://doi.org/10.2337/db18-0927.