Key takeaways:
~ Choline is an essential nutrient that your body uses in several important ways.
~ Choline is needed for brain function and cellular health. It can also be utilized in the methylation cycle as a methyl donor.
~ Genetic variants can impact how well you create choline or how you convert between types of choline.
Choline: from nootropics to gut health
Choline is a vitamin-like nutrient; it’s incorporated and used in every cell of your body. Like many essential biological processes, there are redundant pathways for ensuring choline is available. But without choline in the diet, these synthesis pathways can’t keep up. Choline deficiency can cause everything from cognitive problems to fatty liver disease.
What do the different types of choline do in the body?
Choline is absorbed in different forms from your diet and converts into the following forms.[ref]
- Betaine, an oxidized form of choline, can be used as a methyl donor.
- Acetylcholine is an acetylated form of choline used extensively as a neurotransmitter, essential in learning, attention, and memory.
- Phosphatidylcholine, the phosphorylated form, is incorporated into your cell membranes to make them more fluid.
Tradeoffs to consider:
Choline is necessary in the right amount. Not enough choline impacts cognitive function, reproduction, and liver function. Too much choline, though, can interact with the gut microbiome to produce a choline-derived metabolite (TMAO), which is linked to an increased risk of heart disease.[ref]
Making choline endogenously (in the body):
Humans can make choline endogenously but not at sufficient levels to meet all of our needs. This is why choline is considered an essential nutrient — we need to get at least part of our daily requirement from foods.
In the liver, the PEMT enzyme can convert phosphatidylethanolamine into phosphatidylcholine (PC) with the addition of a methyl group.
Choline creation in the body depends both on having enough of the PEMT enzyme (genetics) as well as sufficient methyl groups (diet, genetics) along with methionine (amino acid, abundant in high protein foods).
Here’s an overview of the biochemical pathways for choline creation. The genes involved are in rectangular boxes.
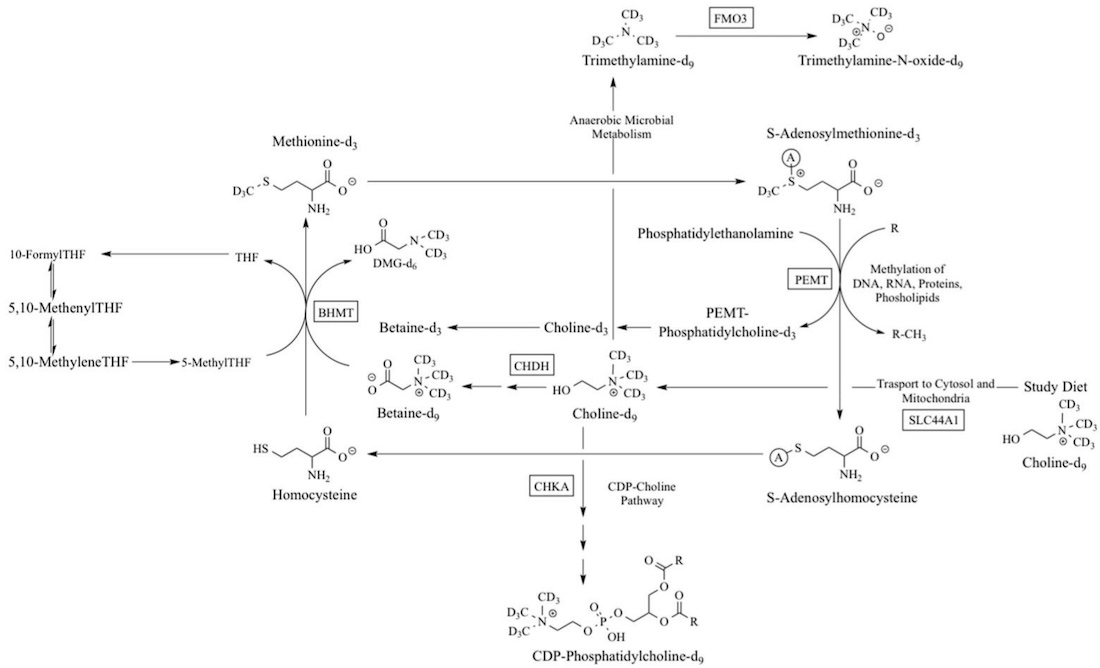
Choline recommendations:
The daily recommended adequate intake of choline is 550 mg for men and 425mg for women.
The AI for choline may not be enough for everyone, though. In one study, some men developed signs of liver damage when restricted to only 550 mg/day of choline.[ref] Other studies point to genetic variants and ancestry as impacting the dietary requirement for choline.[ref]
The Choline Information Council states that 90% of people in the US are choline deficient.
How quickly can you run out of choline?
A research study in the early 90s had healthy male volunteers eat a diet containing no choline for three weeks along with adequate, but not excess, methionine. (The liver can make some choline with methionine.) The results showed “plasma choline and phosphatidylcholine concentrations decreased an average of 30% during the 3-week period when a choline-deficient diet was ingested”. Liver enzyme tests showed that there were signs of liver dysfunction beginning.[ref]
A follow-up study was done with both men and women on a low-choline (50 mg/day) diet. The study lasted either 42 days or until signs of organ failure, whichever came first!
The results showed that men and postmenopausal women were more likely to have organ damage faster than premenopausal women, indicating that estrogen may play a role in how the body prioritizes choline creation.[ref]
Indeed, later research shows that an estrogen response element directly affects PEMT expression.[ref] Thus, women who are premenopausal induce PEMT and create choline at higher levels. This is likely due to choline being essential in reproduction. It protects the fetus if the mother’s diet lacks choline, which was a situation often faced by our ancestors with seasonal deficiencies.
Choline as a nootropic:
When you look at supplemental choline products, such as citicoline or Alpha-GPC, you’ll find that some are marketed as nootropics that supposedly increase focus and memory. Sounds great, but how does this work?
Let’s dig into what choline is doing in the brain and elsewhere…
Acetylcholine is chemically defined as an ester-containing acetic acid and choline. Acetylcholine acts as a neurotransmitter in the brain- signaling from one neuron to another. There are several different neurotransmitters in the brain, and their activation of different types of neurons causes different actions.
Acetylcholine-activated brain regions are important in attention, memory, arousal, and motivation.[ref]
The parasympathetic nervous system uses acetylcholine almost exclusively as the neurotransmitter. The parasympathetic nervous system is responsible for the autonomic actions that you don’t have to think about – digestion, salivation, urination, tears, eye movement, and heart rhythm (at rest). Motor neurons also use acetylcholine to activate muscles. Thus, acetylcholine touches on a lot of different systems in the body.
When looking at changes in the brain from choline, researchers use animal models to trace what is happening.
Animal studies show that choline, specifically Alpha-GPC, potentiates the release of neurotransmitters in the hippocampus. Alpha-GPC also increased striatal dopamine levels, likely by increasing acetylcholine availability.[ref][ref]
Both CDP-choline (citicoline) and Alpha-GPC increase acetylcholine transporters and acetylcholine synthesis in the brain.[ref]
My takeaway: For people who don’t get enough choline in their diets (90% of the population), either citicoline or alpha-GPC may help a bit with memory and focus.
The scoop on TMAO:
I mentioned above that the drawback to higher choline intake is the creation of TMAO.
Trimethylamine N-oxide (TMAO) is a molecule generated by gut bacteria in the intestines from choline, betaine, and carnitine.
Recently, a bunch of studies have been published that correlate high TMAO levels to heart disease and possibly neurological disorders. But correlation isn’t the same as causation, so do you need to worry about TMAO?
Let’s look at how TMAO is made…
In the gut, bacteria can turn choline, betaine, L-carnitine, ergothioneine (from mushrooms), and other choline-containing compounds into trimethylamine N-oxide (TMA). Additionally, TMA and TMAO can be found in fish.
TMA from food and gut bacteria is rapidly absorbed in the intestines and travels to the liver. The FMO3 enzyme converts TMA to TMAO.
Related article: FMO3 genetic variants
Factors influencing TMAO formation include age, diet, and the gut microbiome. TMAO levels tend to increase as we age. FMO3 activity is impacted by diet, with Brassica vegetables, such as cabbage or broccoli, decreasing FMO3 and thus TMAO. The gut microbiome, of course, affects TMA production, and the amount of fiber in the diet can alter the gut microbiome.[ref]
In general, older people and people who eat a typical, high-fat Western diet have higher levels of TMAO. And are more likely to have atherosclerosis and heart disease.
The question of how or if high TMAO causes heart disease is still being studied. While the two are clearly linked, it is harder to prove that TMAO causes heart attacks. For example, TMAO levels are higher in people with diabetes, metabolic syndrome, or kidney problems. All of these are also risk factors for heart disease. One study followed 339 coronary artery disease patients for eight years. The results showed that TMAO levels are higher in people with diabetes, metabolic syndrome, or kidney problems. All of these are confounders. Interestingly, the study didn’t show that TMAO or betaine levels were associated with heart attacks. The study concluded, “Plasma levels of TMAO are confounded by impaired kidney function and poor metabolic control but are not associated with the history, presence or incidence of symptoms or events of coronary heart disease.”[ref]
Genetic studies also show that diabetes and kidney disease-related genetic variants may be causing the increase in TMAO, further confounding the correlation between TMAO and cardiovascular disease.[ref]
On the other hand, animal studies show a link between TMAO and heart-related tests. In mice, TMAO levels correlated with heart dysfunction. Moreover, blocking the formation of TMAO while keeping the same diet prevented cardiac dysfunction.[ref]
Cell studies show that TMAO increases vascular cell adhesion molecule-1, which is implicated in atherosclerosis.[ref]
A recent study on supplemental choline, alpha-GPC, found that there was a correlation between stroke risk and taking alpha-GPC.[ref]
While there is still a lot of research needed here, to err on the side of caution, you likely want to avoid TMAO being high.
Choline Genotype Report
Members: Log in to see your data below.
Not a member? Join here.
Why is this section is now only for members? Here’s why…
Member Content:
Why join Genetic Lifehacks?
~ Membership supports Genetic Lifehack's goal of explaining the latest health and genetics research.
~ It gives you access to the full article, including the Genotype and Lifehacks sections.
~ You'll see your genetic data in the articles and reports.
Join Here
Lifehacks:
All of this information about how the body uses choline is great, but I bet you are now wondering “how do I use it?”.
Diet or Supplements?
With 90% of people in the US not meeting the minimal intake of choline, you may be wondering if everyone needs a choline supplement.
Foods high in choline include:
- liver
- eggs
- bacon
- beef
- pork
- soybeans
- wheat germ
Related article: Choline-rich foods and recipes
First, figure out how much choline you consume on average. Cronometer.com is a free web app for tracking micro and macronutrients. Choline isn’t included in the standard view, but you can go into the settings and include it. Track your food for a few days and see what you consume. For example, if you’re eating liver regularly, you are likely getting enough choline, but for liver-haters, it can be tough.
For most populations, eggs are the primary dietary source of choline. High-fiber bread (wheat germ) is the main dietary source of betaine.[ref]
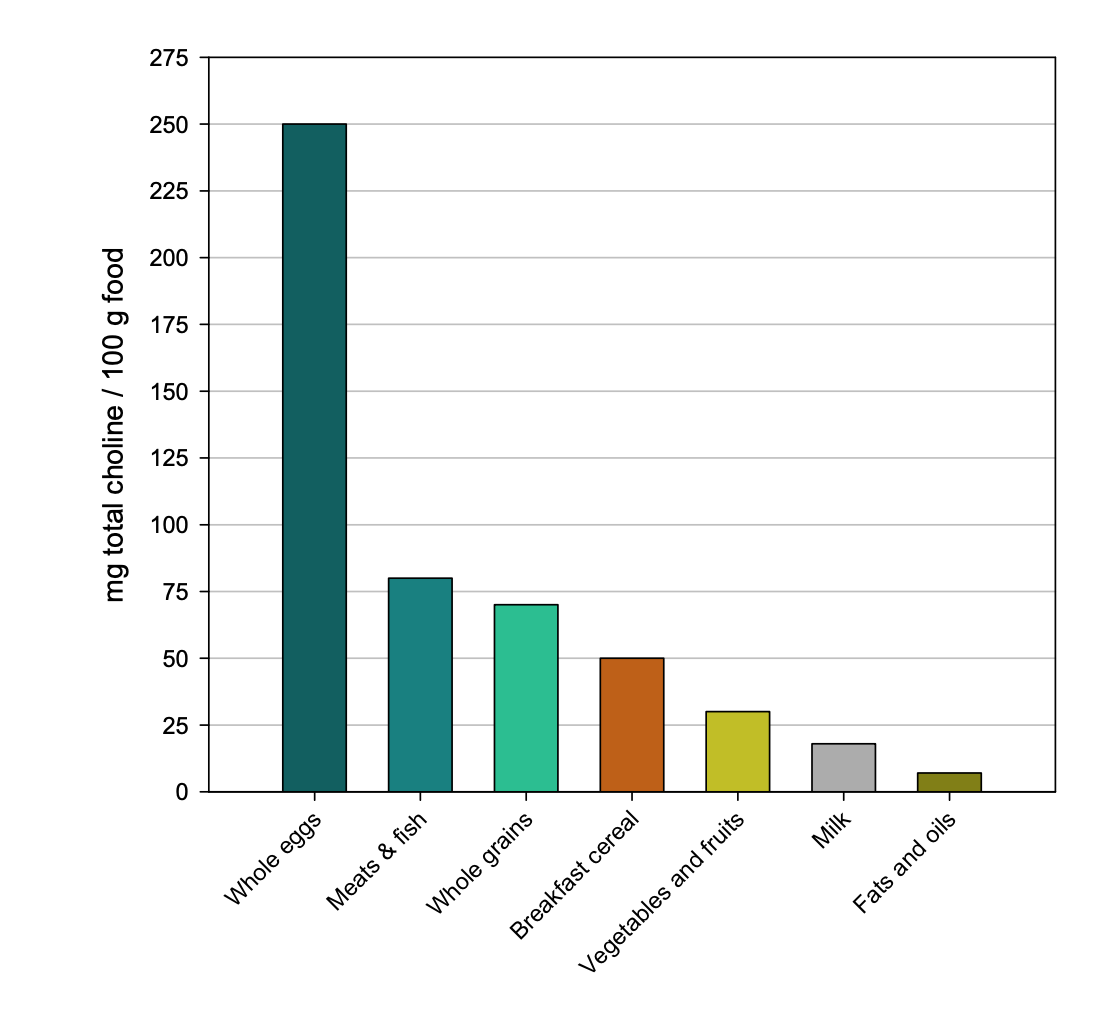
Eggs are better: A research study looked at choline uptake from foods vs. supplemental choline bitartrate. The plasma choline response was four times higher after consuming egg yolks with 3g of choline compared with a choline bitartrate supplement with 3g of choline.[ref]
Another study looked at betaine supplementation vs. betaine and/or choline from foods. All increased choline levels and decreased homocysteine.[ref]
TMAO considerations:
Testing: If you have concerns about heart disease and are worried that choline will make it worse, you could get a TMAO test done to know your current levels. If you are seeing a cardiologist, they may order the test for you. If not, you can order one yourself for around $100. UltaLab Tests is one place to order the test, but you’ll have to get a blood draw done somewhere. Check around for the best prices when ordering lab tests online – test prices vary a lot, and most ordering sites offer coupons and sales. For example, UltaLab Tests usually has a coupon on the home page.
Resveratrol: A clinical trial shows that a polyphenol formula with resveratrol decreased TMAO levels by 62% in 4 weeks.[ref]
Upper limit of choline: Large doses of choline not only can increase TMAO, but it can also result in a fishy, odd-smelling body odor. The tolerable upper intake level for choline is set at 3,500 mg/day for adults.[ref]
Types of Supplemental Choline:
Types of choline supplements include:
- CDP-choline (Citicoline)
- Alpha-GPC choline
- phosphatidylcholine
- betaine (TMG, trimethylglycine)
- choline bitartrate
Things to consider when choosing supplemental choline include the conversion to TMAO, the absorption and utilization, and the source. Many phosphatidylcholine supplements are made from soy, which some people want to avoid.
Citicoline
The rest of this article is for Genetic Lifehacks members only. Consider joining today to read about the types of choline supplements, supplement stacks that use choline, high-choline foods, and a recap of your genetic data with supplement options.
Member Content:
Why join Genetic Lifehacks?
~ Membership supports Genetic Lifehack's goal of explaining the latest health and genetics research.
~ It gives you access to the full article, including the Genotype and Lifehacks sections.
~ You'll see your genetic data in the articles and reports.
Join Here