Good blood glucose regulation is an important factor in overall health and longevity. High blood glucose levels, whether after a meal or all the time, can increase oxidative stress in the body, leading to long-term chronic health problems.
Genetics plays a big role in your blood glucose regulation. Some people may be able to get away with eating junk food and not exercising as much as they should. But for others, genetic sensitivity combines with poor food choices to elevate blood glucose levels. Members will see their genotype report below, plus additional solutions in the Lifehacks section. Join today.
Genetics and blood glucose regulation:
Before we get into the genes involved in regulating blood glucose levels, let’s cover the basics of how the body regulates blood sugar levels. (Skip ahead if you already understand all this.)
Your body regulates the amount of glucose in the blood through a complex system of negative feedback loops. You don’t want to have super high blood sugar levels and you also don’t want low blood sugar levels.
- When blood sugar rises after you eat, your body regulates it to come back to a normal level.
- Likewise, when blood sugar levels fall because you haven’t eaten, your body brings it back up to a normal level.
Two main hormones are essential for maintaining glucose levels in a narrow range: insulin and glucagon.
Insulin and glucagon:
Insulin is released by beta cells in the pancreas in response to high glucose levels in the bloodstream. While usually constant, low-level insulin is available, when glucose levels rise, insulin is released in high enough amounts to counteract the higher blood glucose.
In response to insulin, cells in the skeletal muscle, adipose (fat) tissue, and red blood cells increase their absorption of glucose.
In addition to insulin, pancreatic beta cells also release amylin, which slows gastric emptying and tells your brain that you are full. This slowing of digestion keeps blood glucose levels from spiking too quickly after you eat.
The other hormone involved here is glucagon. The alpha cells of the pancreas secrete glucagon. But it acts in the opposite direction from insulin — when blood glucose levels are low, glucagon is secreted.
Glucagon signals to the liver to convert glycogen into glucose and release it into the bloodstream. If needed, glucagon can also stimulate the liver and muscle cells to create glucose from amino acids through a process called gluconeogenesis.
Regulating blood glucose levels:
The normal range for blood glucose is usually defined as between 65 and 100 mg/dl when you haven’t eaten for a while – the fasted state. (This range varies a little bit, depending on who is defining normal). Within two to three hours of a meal, blood glucose levels of under 180 mg/dl are considered OK.
The release of insulin and glucagon is a negative feedback loop: when blood glucose is high, insulin is released, inhibiting the release of glucagon. When blood glucose levels drop, glucagon is released, and insulin is inhibited.
This system becomes dysregulated in diabetes. People with diabetes may have problems creating enough insulin well, as glucagon still causes the liver to release some glucose. Thus blood glucose levels rise.
While the obvious solution to high blood glucose levels is to decrease carbohydrate intake, it isn’t always that simple – nor is it the right answer for everyone. The genotype report section below goes into additional ways to decrease blood glucose levels that are individualized for your genes.
Another way to quantify your blood sugar levels is to look at your A1c results. Testing HbA1c gives you an average of glucose levels over a few months. According to the American Diabetes Association, an A1C level between 5.7 and 6.5%is in the prediabetes range, while over 6.5% is in the diabetes range.[ref]
Related article: Genes that Impact A1c Levels
Why should you worry about blood glucose levels or insulin sensitivity if you don’t have diabetes?
Glucose is tightly regulated by the body because it is toxic at higher levels. You’ve gotta have it, but don’t want too much. (Yes, you can burn fatty acids for fuel also, but the brain requires some glucose.)
Doctors start talking about diabetes when fasting blood glucose levels are above 125 mg/dL and an oral glucose tolerance test result of above 200 mg/dL.[ref]
If your fasting blood glucose is in the 100-125 mg/dL range for a few tests, then you are likely to be labeled as pre-diabetic.
But what if, once in a while, your blood sugar is high after eating sweets? Or what if your fasting glucose is always around 99 mg/dL, just under the pre-diabetes range? As you can see, there are a lot of gray areas here.
There are three ways that higher blood glucose concentrations are bad[ref]:
- Damage due to osmosis: High glucose causes dehydration and excessive urination. Glucose causes an osmotic reaction – moving water from one side of a membrane to another. Hyperglycemia (really high blood sugar) can be deadly.
- Increased oxidative stress: Glucose that isn’t used for energy can participate in reactions that cause the increased formation of reactive oxygen species (ROS). This increased ROS is what causes long-term damage, such as kidney damage or heart problems, in people with diabetes.[ref]
- Advanced glycation end products: Excess glucose can form a complex with certain other molecules to form advanced glycation end products. This can increase the risk of heart disease and other chronic diseases.
Thus, consistently higher blood glucose levels, even if not in the pre-diabetic/ diabetic range, can increase oxidative stress and the formation of advanced glycation end products.
T-Cell Response:
One more important point here is that insulin and insulin sensitivity are essential for T-cells in the immune system to fight off viruses. People who have problems with insulin release (such as in diabetes) or with insulin sensitivity (insulin resistance, prediabetes) do not have as robust of a T-cell response to viruses.[ref]
Let’s dig deeper into the blood glucose regulation system and take a look at some of the genes involved and the solution for those genes.
Which genes are controlling blood glucose levels?
By looking at the genetic variants that significantly impact blood glucose or insulin levels, we can better understand the control mechanisms in place for this complicated feedback loop.
Understanding where exactly your genetic susceptibility to blood sugar problems lies can help you to figure out a targeted plan for keeping glucose levels stable.
I’m going to break this down into sections, but realize that all of this works together:
- regulating insulin release
- taking glucose into cells (insulin resistance)
- glucagon and regulating glucose release from the liver
- digestive system response to food (GIP and GLP-1)
- overnight regulation of insulin (melatonin in the pancreas)
We will take a look at the science first to understand how the genes work, and then you can check your genes in the Genetic Variants section below (with the specific Lifehacks included).
#1 – Regulating insulin release:
KCNJ11 gene: The KCNJ11 gene encodes a potassium channel subunit found in the beta cells of the pancreas. This ATP-sensitive channel opens and closes in response to blood glucose levels. When glucose is present, ATP levels rise (cells use glucose to make more ATP). When ATP rises, the ATP-sensitive potassium channel in pancreatic beta cells closes, which then triggers the release of insulin.[ref]
Genetic variants in KCNJ11 can decrease the beta cell’s insulin response to blood glucose.
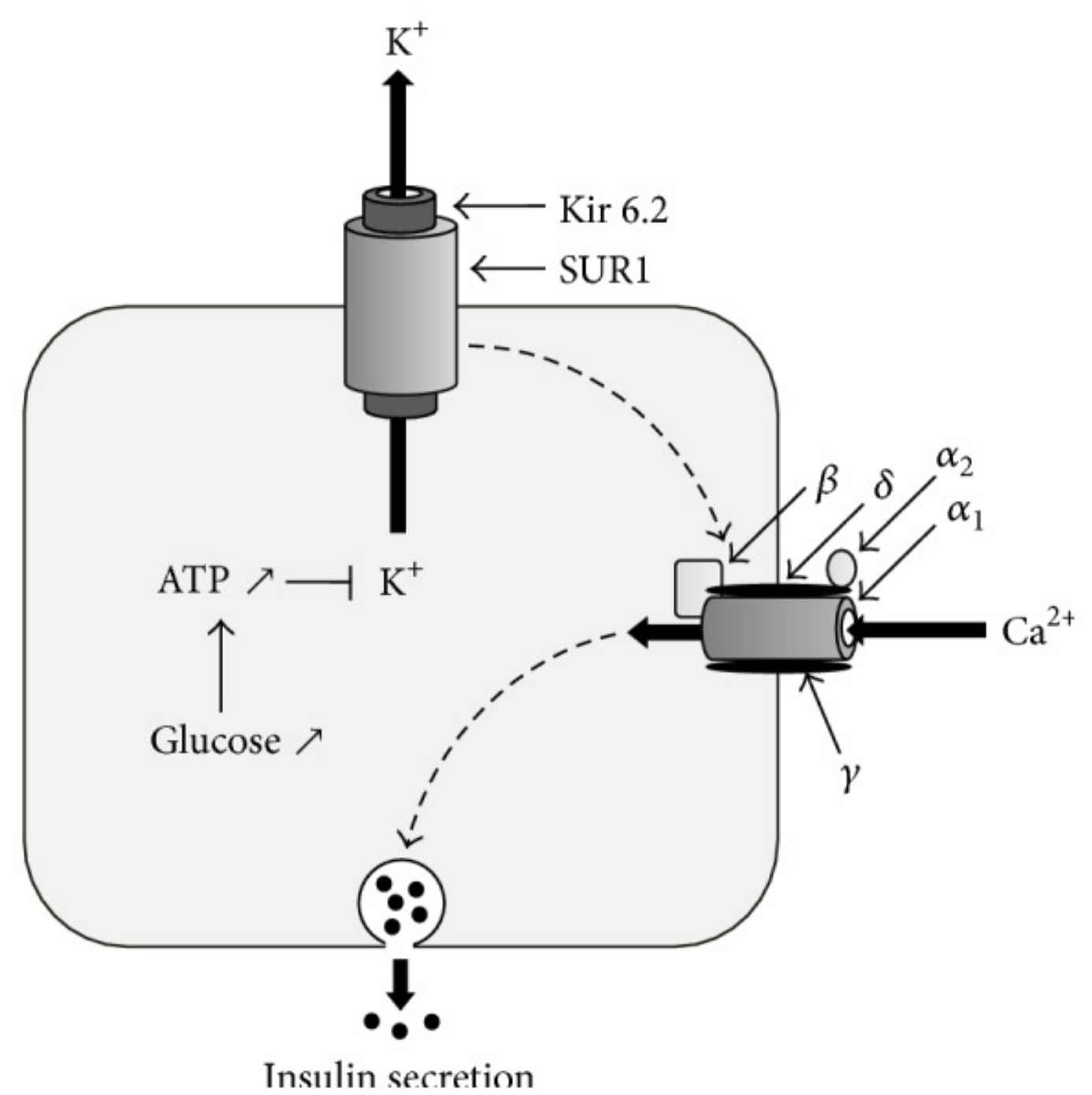
ABCC8 gene: The KCNJ11 gene (above) encodes one subunit of the ATP-sensitive potassium channel. Another subunit, known as SUR1, is encoded by the ABCC8 gene.
The type of diabetes drugs called sulfonylureas bind to SUR1 and thus increase insulin release. Genetic variants in ABCC8 can impact blood glucose levels.
Glucokinase (GCK gene) is important in regulating glucose metabolism in the liver and in beta-cells. In the beta cells, glucose can come in via the GLUT2 receptor (which doesn’t need insulin). Glucokinase in the pancreas can amplify the signal from rising glucose levels, increasing insulin secretion. Genetic variants in the GCK gene are one risk factor for diabetes.
CDKAL1 (Cyclin-dependent kinase 5 regulatory subunit associated protein 1-like 1) is part of the signaling pathway that causes insulin release. Genetic variants in this gene can cause decreased insulin release, which then keeps blood glucose levels higher when eating carbs/sugar.[ref][ref]
#2 – Taking up glucose into cells (insulin resistance genes):
Glucose molecules are too big to cross the cell membrane without assistance. Thus, for glucose to get into a cell, it needs to be transported. There are actually several different glucose transport methods, depending on tissue type.
Muscles use a lot of energy, and transporting glucose into the muscle is a way the body regulates blood glucose levels.
In muscle tissue, GLUT4 (glucose transporter 4) transports glucose into the cells. The GLUT4 transporter is located inside the cells, and it needs to be translocated to the cell membrane in order to move in glucose.
Insulin, binding to an insulin receptor on the cell, causes a signal to be sent to the GLUT4 receptor, moving it to the cell membrane. This results in glucose being taken up by the cell via the GLUT4 receptor.
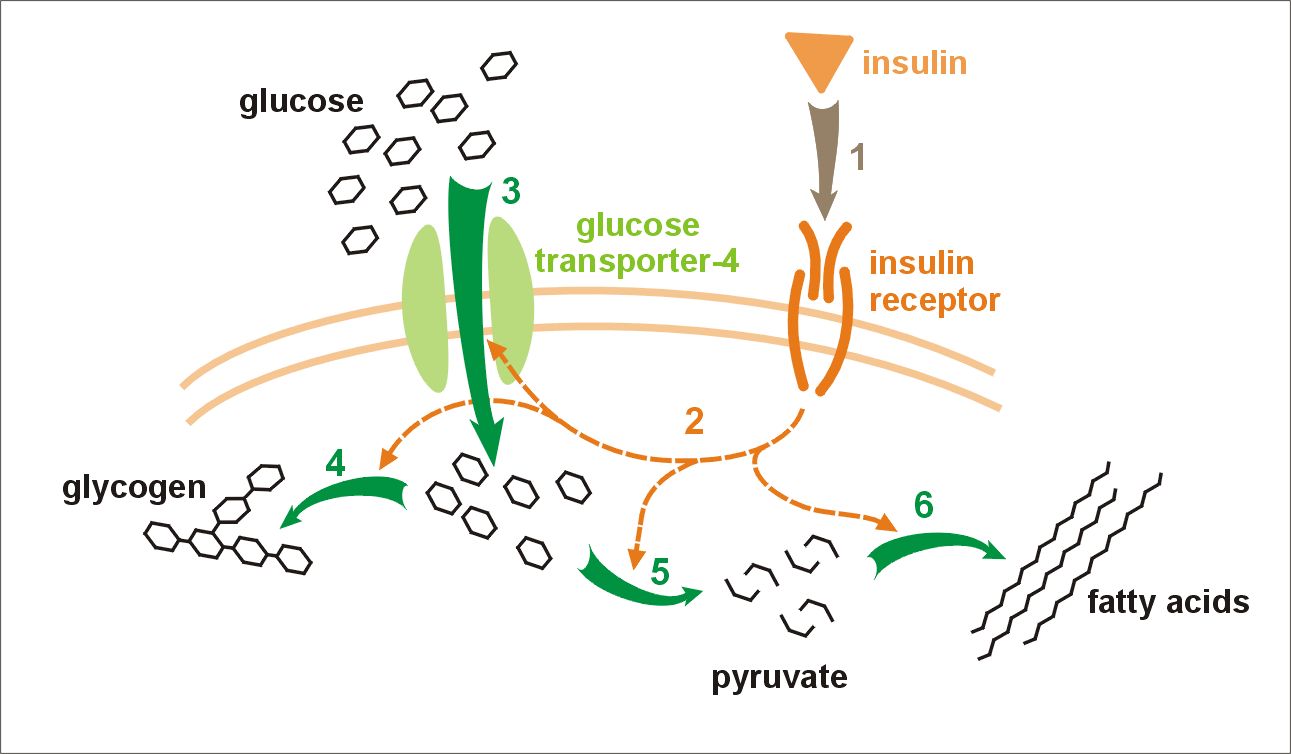
There are several genes involved in the insulin receptor and the secondary signals sent to GLUT4. The main insulin receptor gene, INSR, is essential for life, and thus mutations here are rarely compatible with life. But variants in the signals generated by insulin binding to the insulin receptor can change the way that the signal is sent to the GLUT4 receptor.
The IRS1 (insulin receptor substrate 1) gene codes for a key protein in the insulin-stimulated signal pathway. After insulin binds to the insulin receptor, IRS1 is one of the molecules activated to send the message. Variants in IRS1 also have links to an increase in the risk of type-2 diabetes.[ref]
Another gene that interacts with the insulin receptor is ENPP1 (ectoenzyme nucleotide pyrophosphate phosphodiesterase 1). This enzyme downregulates the signal from insulin by interacting with one of the insulin receptor subunits. Genetic variants in this gene are associated with insulin resistance.[ref]
In addition to insulin signaling for GLUT4 to move to the cell membrane, exercise also causes GLUT4 to translocate and take in glucose.[ref]
#3 – Genes involved with glucogen: glucose release from the liver
The other half of the picture with glucose regulation is the signal to the liver to stop turning glycogen into glucose and releasing it into the bloodstream.
The alpha cells in the pancreas release glucagon when blood sugar levels drop. Glucagon signals the liver to turn glycogen into glucose and then release it, which is called glycogenolysis (-lysis = breaking up, so breaking up glycogen).
Lower blood glucose in the alpha-cells causes a decrease in ATP production, which subsequently changes the polarization of certain ion channels. This change in electrical potential then causes calcium to come into the cells, triggering the release of glucagon.[ref]
The KCNH2 gene codes for a potassium-ion channel that has several different roles in the body. It is important in the heart in regulating rhythm, and it has recently been discovered to play an important role in the alpha-cells of the pancreas with the release of glucagon.[ref] Genetic variants in KCNH2 have links to a decrease in glucagon production.
#4 – Genetics and GLP-1:
There are a couple of other important hormones that come into play with glucose regulation as well.
GIP (glucose-dependent insulinotropic peptide) and GLP-1 (glucagon-like peptide-1) are released by cells at the beginning of the intestines in response to food.
While glucagon increases blood glucose levels via stimulating the liver to release glucose, glucagon-like peptide-1 (GLP-1) has pretty much the opposite effect in that it can decrease blood glucose by stimulating more insulin to be released by the beta-cells in the pancreas.[ref]
GIP (glucose-dependent insulinotropic peptide) not only acts on the GIP receptor (GIPR) in the pancreas, but it also acts upon other tissues such as fat, bone, and endothelial cells lining blood vessels.
GLP-1 has multiple functions as well. Acting on the GLP-1 receptor in various tissues, GLP-1 reduces appetite, stimulates insulin synthesis, and promotes bone formation.
GLP1 levels are impacted by variants in the SLC5A1 gene, which encodes the sodium/glucose cotransporter 1 (SGLT1). SGLT1 is the transporter in the intestinal cells that allows for the uptake of glucose from the foods you’ve eaten.
TCF7L2: One of the first genes identified as a risk factor for diabetes was TCF7L2, but initially, researchers weren’t sure why genetic variants in this gene increased the risk of diabetes.
More than a decade of research now shows that TCF7L2, a transcription factor regulator, impacts the production of insulin in the pancreas. While there are still many questions about TCF7L2 left unanswered, it has been shown that TCF7L2 is essential to the production of proglucagon via the intestinal cells (not the glucagon produced in the alpha cells of the pancreas).[ref]
Genetic variants in TCF7L2 are associated with impaired glucose stimulation of insulin secretion and GLP-1.[ref]
GLP1R (glucagon-like peptide 1 receptor) receives the signal from GLP-1, and variants in GLP1R have associations with altered BMI, weight, and insulin resistance.
#5 – Overnight blood glucose regulation: melatonin genetic variants
Melatonin is a hormone released in large quantities at night by the pineal gland.
Increased melatonin at night decreases insulin secretion.[ref]
This should be the normal way the body works — you aren’t eating at night, and thus insulin should be suppressed. Think about it: Your ancestors weren’t raiding the refrigerator for a midnight snack because there wasn’t a fridge to raid or a microwave to heat things up. Insulin levels needed to be low while sleeping so that an individual didn’t become hypoglycemic at night.
Genetics comes into play here. Variants in the melatonin receptor found in the beta-cells of the pancreas are linked to diabetes. The MTNR1B (melatonin receptor) variants increase the risk of diabetes — but only in people who are eating dinner late or snacking heavily at night.[ref][ref][ref][ref][ref]
Glucose Regulation Genotype Report
Members: Log in to see your data below.
Not a member? Join here.
Why is this section is now only for members? Here’s why…
What about diabetes-related genes?
I’ve focused here on the variants specifically related to blood glucose regulation. Please also read through the articles on type 2 diabetes genes.
Lifehacks for lowering blood glucose levels:
In addition to the specific gene-related lifehacks above in the genotype report, here are several additional research-based methods for lowering blood glucose levels. Talk with your doctor or health care provider about diabetes medication options if you have consistently high blood glucose.
Blood glucose monitoring:
Obviously, reducing sugar and all carbohydrate intake should decrease blood glucose levels. But it isn’t as necessarily as simple as just looking up the glycemic index of food. That’s a starting point, but a large individual variation exists in how different foods affect blood sugar levels. For example, eating a banana will spike blood glucose levels for some, but for others, it may not have an effect.
A study of 800 people wearing continuous blood glucose monitors showed that individual glucose responses varied widely and depended on many variables, including the gut microbiome, normal dietary habits, and physical activity level.[ref]
The best way to know how foods affect you would be to wear a continuous blood glucose monitor.
Regular, stick-your-finger type of blood glucose meters are available without a prescription, so this is an option for spot-checking to see how certain foods affect your blood sugar. It’s also a great way to know your baseline fasting blood glucose. A poor night’s sleep significantly affects morning glucose levels, so you would need to track for a week or so and average out the readings.
You can also order your own blood tests if you want to do a fasting blood glucose test from a lab, perhaps as a way of double-checking the accuracy of your meter.
Exercise decreases blood glucose levels:
Moderate to intense exercise causes the GLUT4 receptors to translocate to the cell membrane in muscle cells. This increases the uptake of glucose into the muscle cells, and thus lowers blood glucose levels.[ref] (This is also why hard exercise could cause hypoglycemia in people who are prone to low blood sugar.)
Quality of Sleep:
Many studies link poor sleep to higher fasting blood glucose levels. Sleep apnea is a big risk factor for type 2 diabetes. If you have been ignoring your sleep apnea, new options on the market may help- talk with your doctor and make quality sleep a priority.[ref][ref]
In addition to sleep quality, the quantity of sleep is also important. A recent (2019) study showed that 4 hours of sleep significantly reduced insulin sensitivity and impaired beta-cell function (compared to 8 hours of sleep). Moreover, if the sleep loss occurred in the early morning hours (e.g., waking up at 3 am and not falling back to sleep), cortisol levels were also impacted.[ref]
Research-backed Supplements for Blood Glucose Regulation:
Related Articles and Topics:
Top 10 Genes to Check in Your Genetic Raw Data
These are 10 genes with important variants that can have a big impact on health. So check them out, cross them off your list if you don’t have them — and read the articles to learn more if you do carry the variant.
Diabetes: Genetic Risk Report
We often talk about diabetes as though it is one disease, but diabetes can have several different causes or pathways that are impacting glucose regulation. Tailoring your diabetes prevention (or reversal) efforts to fit your genetic susceptibility may be more effective.
Intermittent Fasting: Benefits from changing Gene Expression
What is interesting about IF is that it can change gene expression in different tissues in the body. Something as simple as ‘not eating’ can cause an upregulation of proteins associated with longevity. This article digs into the recent research on intermittent fasting, focusing on how it changes gene expression.
Fatty Liver: Genetic variants that increase the risk of NAFLD
Non-alcoholic fatty liver disease (NAFLD) is now the leading cause of liver problems worldwide, bypassing alcoholic liver disease. The good news is that fatty liver disease is often reversible. Read on for the science details, genetic susceptibility variants, and lifehacks for a healthy liver.